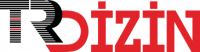
Review: mRNA delivery systems in vaccinations and COVID-19
Yıl: 2022 Cilt: 26 Sayı: 5 Sayfa Aralığı: 1084 - 1101 Metin Dili: İngilizce DOI: 10.29228/jrp.205 İndeks Tarihi: 29-09-2022
Review: mRNA delivery systems in vaccinations and COVID-19
Öz: mRNA vaccines open promising avenues for overcoming a variety of diseases due to their high therapeutic utilities, rapid growth capacities, and safe administration potentials. With the emergence of COVID-19, the use of mRNA vaccines has become even more widespread and far-reaching. However, for mRNA to be delivered to target cells and tissues, several obstacles must be overcome. For instance, naked mRNAs get easily and hastily degraded by ribonucleases in tissues and the bloodstream, and since mRNAs are large and polyanionic molecules, they cannot passively diffuse across cell membranes. Even though mRNAs are internalized by APCs, they must be able to reach the cytoplasm and escape endo-lysosomal traffic. Therefore, distinctive transport systems for efficient encapsulation of mRNAs using nanocarrier systems are required to ensure their delivery to cells’ cytoplasm. At this point, non-viral gene delivery systems such as polymers and lipids come to the fore, in which they can overcome the biological barriers and provide efficient delivery of mRNAs. Recently, mRNA vaccines have been used as a powerful weapon against COVID- 19 pandemic which has affected the whole world since December 2019. This was clear by the emergence of Pfizer- BioNTech and Moderna vaccines, which offered mRNA vaccines with auspicious treatment abilities. A variety of carrying candidates have been utilized in such vaccines as polymers, metal nanoparticles, as well as LNPs, which each comes with its cons and pros in delivering mRNA. All of these mentioned points will be clarified and discussed in detail in this review paper.
Anahtar Kelime: Belge Türü: Makale Makale Türü: Derleme Erişim Türü: Erişime Açık
- [1] Riedel S. Edward Jenner and the history of smallpox and vaccination. Proc (Bayl Univ Med Cent). 2005; 18(1): 21- 5. [CrossRef]
- [2] Younger DS, Younger AP, Guttmacher S. Childhood Vaccination: Implications for Global and Domestic Public Health. Neurol Clin. 2016; 34(4): 1035-47. [CrossRef]
- [3] Standaert B, Rappuoli R. Towards a more comprehensive approach for a total economic assessment of vaccines?: 1. The building blocks for a health economic assessment of vaccination. J Mark Access Health Policy. 2017; 5(1): 1335162. [CrossRef]
- [4] Maruggi G, Zhang C, Li J, Ulmer JB, Yu D. mRNA as a Transformative Technology for Vaccine Development to Control Infectious Diseases. Mol Ther. 2019; 27(4): 757-72. [CrossRef]
- [5] Corey L, Mascola JR, Fauci AS, Collins FS. A strategic approach to COVID-19 vaccine R&D. Science. 2020; 368(6494): 948-50. [CrossRef]
- [6] Fries LF, Gordon DM, Richards RL, Egan JE, Hollingdale MR, Gross M, et al. Liposomal malaria vaccine in humans: a safe and potent adjuvant strategy. Proc Natl Acad Sci U S A. 1992; 89(1): 358-62. [CrossRef]
- [7] Salomon N, Vascotto F, Selmi A, Vormehr M, Quinkhardt J, Bukur T, et al. A liposomal RNA vaccine inducing neoantigen-specific CD4(+) T cells augments the antitumor activity of local radiotherapy in mice. Oncoimmunology. 2020; 9(1): 1771925. [CrossRef]
- [8] Chen X, Han W, Wang G, Zhao X. Application prospect of polysaccharides in the development of anti-novel coronavirus drugs and vaccines. Int J Biol Macromol. 2020; 164: 331-43. [CrossRef]
- [9] Dykman LA. Gold nanoparticles for preparation of antibodies and vaccines against infectious diseases. Expert Rev Vaccines. 2020; 19(5): 465-77. [CrossRef]
- [10] Nevagi RJ, Skwarczynski M, Toth I. Polymers for subunit vaccine delivery. Eur Polym J. 2019; 114: 397-410. [CrossRef]
- [11] Lambricht L, Peres C, Florindo H, Préat V, Vandermeulen G. Polymer-based nanoparticles as modern vaccine delivery systems. Micro Nanotechnol Vaccine Dev. 2017: 185-203. [CrossRef]
- [12] Ganda IS, Zhong Q, Hali M, Albuquerque RL, Padilha FF, da Rocha SR, et al. Dendrimer-conjugated peptide vaccine enhances clearance of Chlamydia trachomatis genital infection. Int J Pharm. 2017; 527(1-2): 79-91. [CrossRef]
- [13] Igyártó BZ, Jacobsen S, Ndeupen S. Future considerations for the mRNA-lipid nanoparticle vaccine platform. Curr Opin Virol. 2021; 48: 65-72. [CrossRef]
- [14] Thi TTH, Suys EJ, Lee JS, Nguyen DH, Park KD, Truong NP. Lipid-based nanoparticles in the clinic and clinical trials: from cancer nanomedicine to COVID-19 vaccines. Vaccines. 2021; 9(4): 359. [CrossRef]
- [15] Ji Q, Wang S, Ma J, Liu Q. A review: Progress in the development of fish Vibrio spp. vaccines. Immunol Lett. 2020; 226: 46-54. [CrossRef]
- [16] Shin MD, Shukla S, Chung YH, Beiss V, Chan SK, Ortega-Rivera OA, et al. COVID-19 vaccine development and a potential nanomaterial path forward. Nat Nanotechnol. 2020; 15(8): 646-55. [CrossRef]
- [17] Larocca C, Schlom J. Viral vector–based therapeutic cancer vaccines. Cancer J. 2011; 17(5): 359. [CrossRef]
- [18] Li YD, Chi WY, Su JH, Ferrall L, Hung CF, Wu TC. Coronavirus vaccine development: from SARS and MERS to COVID-19. J Biomed Sci. 2020; 27(1): 1-23. [CrossRef]
- [19] Wang M, Jiang S, Wang Y. Recent advances in the production of recombinant subunit vaccines in Pichia pastoris. Bioengineered. 2016; 7(3): 155-65. [CrossRef]
- [20] Oliver SE, Gargano JW, Scobie H, Wallace M, Hadler SC, Leung J, et al. The advisory committee on immunization practices’ interim recommendation for use of Janssen COVID-19 vaccine—United States, February 2021. MMWR Morb Mortal Wkly Rep. 2021; 70(9): 329. [CrossRef]
- [21] Wise J. Covid-19: European countries suspend use of Oxford-AstraZeneca vaccine after reports of blood clots. BMJ; 2021. [CrossRef]
- [22] Bill RM. Recombinant protein subunit vaccine synthesis in microbes: a role for yeast? J Pharm Pharmacol. 2015; 67(3): 319-28. [CrossRef]
- [23] Tan M, Jiang X. Recent advancements in combination subunit vaccine development. Hum Vaccin Immunother. 2017; 13(1): 180-5. [CrossRef]
- [24] VaccinesWork, What are protein subunit vaccines and how could they be used against COVID-19? https://www.gavi.org/vaccineswork/what-are-protein-subunit-vaccines-and-how-could-they-be-used-againstcovid- 19. (accessed April 21, 2022).
- [25] Keech C, Albert G, Cho I, Robertson A, Reed P, Neal S, et al. Patel 495 N, Frieman MB, Haupt RE, Logue J, McGrath M, Weston S, Piedra PA, Desai C, Callahan K, et al. Phase 1–2 Trial 496 of a SARS-CoV-2 Recombinant Spike Protein Nanoparticle Vaccine. N Engl J Med Overseas Ed. 2020; 497: 2320-32. [CrossRef]
- [26] Wadhwa A, Aljabbari A, Lokras A, Foged C, Thakur A. Opportunities and challenges in the delivery of mRNAbased vaccines. Pharmaceutics. 2020; 12(2): 102. [CrossRef]
- [27] Sahin U, Karikó K, Türeci Ö. mRNA-based therapeutics—developing a new class of drugs. Nat Rev Drug Discov. 2014; 13(10): 759-80. [CrossRef]
- [28] Ho W, Gao M, Li F, Li Z, Zhang XQ, Xu X. Next Generation Vaccines: Nanoparticle Mediated DNA and mRNA Delivery. Adv Healthc Mater. 2021; 10(8): 2001812. [CrossRef]
- [29] Schoenmaker L, Witzigmann D, Kulkarni JA, Verbeke R, Kersten G, Jiskoot W, et al. mRNA-lipid nanoparticle COVID-19 vaccines: Structure and stability. Int J Pharm. 2021; 601: 120586. [CrossRef]
- [30] Pardi N, Hogan MJ, Porter FW, Weissman D. mRNA vaccines—a new era in vaccinology. Nat Rev Drug Discov. 2018; 17(4): 261-79. [CrossRef]
- [31] Zhang C, Maruggi G, Shan H, Li J. Advances in mRNA vaccines for infectious diseases. Front Immunol. 2019: 594. [CrossRef]
- [32] Martinon F, Krishnan S, Lenzen G, Magné R, Gomard E, Guillet JG, et al. Induction of virus specific cytotoxic T lymphocytes in vivo by liposome entrapped mRNA. Eur J Immunol. 1993; 23(7): 1719-22. [CrossRef]
- [33] Jirikowski GF, Sanna PP, Maciejewski-Lenoir D, Bloom FE. Reversal of diabetes insipidus in Brattleboro rats: intrahypothalamic injection of vasopressin mRNA. Science. 1992; 255(5047): 996-8. [CrossRef]
- [34] Kowalski PS, Rudra A, Miao L, Anderson DG. Delivering the messenger: advances in technologies for therapeutic mRNA delivery. Mol Ther. 2019; 27(4): 710-28. [CrossRef]
- [35] Sharova LV, Sharov AA, Nedorezov T, Piao Y, Shaik N, Ko MS. Database for mRNA half-life of 19 977 genes obtained by DNA microarray analysis of pluripotent and differentiating mouse embryonic stem cells. Research DNA Res. 2009; 16(1): 45-58. [CrossRef]
- [36] Amor S, Fernández Blanco L, Baker D. Innate immunity during SARS-CoV-2: evasion strategies and activation trigger hypoxia and vascular damage.J Clin Exp Immunol. 2020; 202(2): 193-209. [CrossRef]
- [37] Yang DC, Eldredge AC, Hickey JC, Muradyan H, Guan Z. Multivalent peptide-functionalized bioreducible polymers for cellular delivery of various RNAs. Biomacromolecules. 2020; 21(4): 1613-24. [CrossRef]
- [38] Granot-Matok Y, Kon E, Dammes N, Mechtinger G, Peer D. Therapeutic mRNA delivery to leukocytes. J Control Release. 2019; 305: 165-75. [CrossRef]
- [39] Vallazza B, Petri S, Poleganov MA, Eberle F, Kuhn AN, Sahin U. Recombinant messenger RNA technology and its application in cancer immunotherapy, transcript replacement therapies, pluripotent stem cell induction, and beyond. Wiley Wiley Interdiscip Rev RNA. 2015; 6(5): 471-99. [CrossRef]
- [40] Shu Y, Pi F, Sharma A, Rajabi M, Haque F, Shu D, et al. Stable RNA nanoparticles as potential new generation drugs for cancer therapy. Adv Drug Deliv Rev. 2014; 66: 74-89. [CrossRef]
- [41] Schaffer DV, Koerber JT, Lim K-i. Molecular engineering of viral gene delivery vehicles. Annu Rev Biomed Eng. 2008; 10: 169-94. [CrossRef]
- [42] Seow Y, Wood MJ. Biological gene delivery vehicles: beyond viral vectors. Molecular Therapy. 2009; 17(5): 767-77. [CrossRef]
- [43] Singh N, Liu X, Hulitt J, Jiang S, June CH, Grupp SA, et al. Nature of tumor control by permanently and transiently modified GD2 chimeric antigen receptor T cells in xenograft models of neuroblastoma. Cancer Immunol Res. 2014; 2(11): 1059-70. [CrossRef]
- [44] DiTommaso T, Cole JM, Cassereau L, Buggé JA, Hanson JLS, Bridgen DT, et al. Cell engineering with microfluidic squeezing preserves functionality of primary immune cells in vivo. Proc Natl Acad Sci U S A. 2018; 115(46): E10907- E14. [CrossRef]
- [45] Svoboda J, Rheingold SR, Gill SI, Grupp SA, Lacey SF, Kulikovskaya I, et al. Nonviral RNA chimeric antigen receptor–modified T cells in patients with Hodgkin lymphoma. Blood. 2018; 132(10): 1022-6. [CrossRef]
- [46] Mukalel AJ, Riley RS, Zhang R, Mitchell MJ. Nanoparticles for nucleic acid delivery: Applications in cancer immunotherapy. Cancer Lett. 2019; 458: 102-12. [CrossRef]
- [47] Hajj KA, Whitehead KA. Tools for translation: non-viral materials for therapeutic mRNA delivery. Nat Rev Mater. 2017; 2(10): 1-17. [CrossRef]
- [48] Zhao Y, Huang L. Lipid nanoparticles for gene delivery. Adv Genet. 2014; 88: 13-36. [CrossRef]
- [49] Ghasemiyeh P, Mohammadi-Samani S. Solid lipid nanoparticles and nanostructured lipid carriers as novel drug delivery systems: applications, advantages and disadvantages. Res Pharm Sci. 2018; 13(4): 288. [CrossRef]
- [50] Guevara ML, Persano F, Persano S. Advances in lipid nanoparticles for mRNA-based cancer immunotherapy. Front Chem. 2020: 963. [CrossRef]
- [51] Hassett KJ, Higgins J, Woods A, Levy B, Xia Y, Hsiao CJ, et al. Impact of lipid nanoparticle size on mRNA vaccine immunogenicity. J Control Release. 2021; 335: 237-46. [CrossRef]
- [52] Fan Y-N, Li M, Luo Y-L, Chen Q, Wang L, Zhang H-B, et al. Cationic lipid-assisted nanoparticles for delivery of mRNA cancer vaccine. Biomater Sci. 2018; 6(11): 3009-18. [CrossRef]
- [53] Oberli MA, Reichmuth AM, Dorkin JR, Mitchell MJ, Fenton OS, Jaklenec A, et al. Lipid nanoparticle assisted mRNA delivery for potent cancer immunotherapy. Nano Lett. 2017; 17(3): 1326-35. [CrossRef]
- [54] Bus T, Traeger A, Schubert US. The great escape: how cationic polyplexes overcome the endosomal barrier. J Mater Chem B. 2018; 6(43): 6904-18. [CrossRef]
- [55] SG, Dowdy SF. Overcoming delivery barriers with LNPs. Nat Mater. 2021; 20(5): 575-7. [CrossRef]
- [56] Eygeris Y, Patel S, Jozic A, Sahay G. Deconvoluting lipid nanoparticle structure for messenger RNA delivery. Nano Lett. 2020; 20(6): 4543-9. [CrossRef]
- [57] Thomas SJ, Moreira Jr ED, Kitchin N, Absalon J, Gurtman A, Lockhart S, et al. Safety and efficacy of the BNT162b2 mRNA Covid-19 vaccine through 6 months. N Engl J Med. 2021; 385(19): 1761-73. [CrossRef]
- [58] Bettini E, Locci M. SARS-CoV-2 mRNA vaccines: immunological mechanism and beyond. Vaccines (Basel). 2021; 9(2): 147. [CrossRef]
- [59] Yang T, Li C, Wang X, Zhao D, Zhang M, Cao H, et al. Efficient hepatic delivery and protein expression enabled by optimized mRNA and ionizable lipid nanoparticle. Bioact Mater. 2020; 5(4): 1053-61. [CrossRef]
- [60] Billingsley MM, Singh N, Ravikumar P, Zhang R, June CH, Mitchell MJ. Ionizable lipid nanoparticle-mediated mRNA delivery for human CAR T cell engineering. Nano Lett. 2020; 20(3): 1578-89. [CrossRef]
- [61] Brown CE, Alizadeh D, Starr R, Weng L, Wagner JR, Naranjo A, et al. Regression of glioblastoma after chimeric antigen receptor T-cell therapy. N Engl J Med. 2016; 375(26): 2561-9. [CrossRef]
- [62] Chen S, Tam YYC, Lin PJ, Sung MM, Tam YK, Cullis PR. Influence of particle size on the in vivo potency of lipid nanoparticle formulations of siRNA. J Control Release. 2016; 235: 236-44. [CrossRef]
- [63] Belliveau NM, Huft J, Lin PJ, Chen S, Leung AK, Leaver TJ, et al. Microfluidic synthesis of highly potent limit-size lipid nanoparticles for in vivo delivery of siRNA. Mol Ther - Nucleic Acids. 2012; 1: e37. [CrossRef]
- [64] Yanez Arteta M, Kjellman T, Bartesaghi S, Wallin S, Wu X, Kvist AJ, et al. Successful reprogramming of cellular protein production through mRNA delivered by functionalized lipid nanoparticles. Proc Natl Acad Sci U S A. 2018; 115(15): E3351-E60. [CrossRef]
- [65] Bouchkouj N, Kasamon YL, de Claro RA, George B, Lin X, Lee S, et al. FDA approval summary: axicabtagene ciloleucel for relapsed or refractory large B-cell lymphoma. Clin Cancer Res. 2019; 25(6): 1702-8. [CrossRef]
- [66] Finn JD, Smith AR, Patel MC, Shaw L, Youniss MR, van Heteren J, et al. A single administration of CRISPR/Cas9 lipid nanoparticles achieves robust and persistent in vivo genome editing. Cell Rep. 2018; 22(9): 2227-35. [CrossRef]
- [67] Kowalski PS, Capasso Palmiero U, Huang Y, Rudra A, Langer R, Anderson DG. Ionizable amino polyesters synthesized via ring opening polymerization of tertiary amino alcohols for tissue selective mRNA delivery. Adv Mater. 2018; 30(34): 1801151. [CrossRef]
- [68] Fornaguera C, Guerra Rebollo M, Angel Lazaro M, Castells Sala C, Meca Cortés O, Ramos Pérez V, et al. mRNA Delivery System for Targeting Antigen Presenting Cells In Vivo. Adv Heal Mater. 2018; 7(17): 1800335. [CrossRef]
- [69] Wahane A, Waghmode A, Kapphahn A, Dhuri K, Gupta A, Bahal R. Role of lipid-based and polymer-based nonviral vectors in nucleic acid delivery for next-generation gene therapy. Molecules. 2020; 25(12): 2866. [CrossRef]
- [70] Ulkoski D, Bak A, Wilson JT, Krishnamurthy VR. Recent advances in polymeric materials for the delivery of RNA therapeutics. Expert Opin Drug Deliv. 2019; 16(11): 1149-67. [CrossRef]
- [71] Juanes M, Creese O, Fernández-Trillo P, Montenegro J. Messenger RNA delivery by hydrazone-activated polymers. Medchemcomm. 2019; 10(7): 1138-44. [CrossRef]
- [72] Ulkoski D, Munson MJ, Jacobson ME, Palmer CR, Carson CS, Sabirsh A, et al. High-throughput automation of endosomolytic polymers for mrna delivery. ACS Appl Bio Mater. 2021; 4(2): 1640-54. [CrossRef]
- [73] Jarzębińska A, Pasewald T, Lambrecht J, Mykhaylyk O, Kümmerling L, Beck P, et al. A single methylene group in oligoalkylamine based cationic polymers and lipids promotes enhanced mRNA delivery. Angew Chem Int Ed Engl. 2016; 55(33): 9591-5. [CrossRef]
- [74] Shetti NP, Mishra A, Bukkitgar SD, Basu S, Narang J, Raghava Reddy K, et al. Conventional and nanotechnologybased sensing methods for SARS coronavirus (2019-nCoV). ACS Appl Bio Mater. 2021; 4(2): 1178-90. [CrossRef]
- [75] WHO Coronavirus (COVID-19) Dashboard. https://covid19.who.int/. (accessed March 15, 2022).
- [76] Kobayashi T, Jung S-m, Linton NM, Kinoshita R, Hayashi K, Miyama T, et al. Communicating the risk of death from novel coronavirus disease (COVID-19). J Clin Med. 2020; 9(2): 580. [CrossRef]
- [77] Anderson RM, Heesterbeek H, Klinkenberg D, Hollingsworth TD. How will country-based mitigation measures influence the course of the COVID-19 epidemic? The Lancet. 2020; 395(10228): 931-4. [CrossRef]
- [78] Mei L-C, Jin Y, Wang Z, Hao G-F, Yang G-F. Web resources facilitate drug discovery in treatment of COVID-19. Drug Discov Today. 2021; 26(10): 2358-66. [CrossRef]
- [79] Callaway E. The race for coronavirus vaccines: a graphical guide. Nature. 2020; 576-7. [CrossRef]
- [80] FDA, FDA Approves First COVID-19 Vaccine. https://www.fda.gov/news-events/press-announcements/fdaapproves- first-covid-19-vaccine. (accessed November 22, 2021).
- [81] Fehr AR, Perlman S. Coronaviruses: an overview of their replication and pathogenesis. Methods Mol Biol. 2015; 1282: 1-23. [CrossRef]
- [82] Pal M, Berhanu G, Desalegn C, Kandi V. Severe acute respiratory syndrome coronavirus-2 (SARS-CoV-2): an update. Cureus. 2020; 12(3). [CrossRef]
- [83] Wu A, Peng Y, Huang B, Ding X, Wang X, Niu P, et al. Genome composition and divergence of the novel coronavirus (2019-nCoV) originating in China. Cell Host Microbe. 2020; 27(3): 325-8. [CrossRef]
- [84] Corman VM, Muth D, Niemeyer D, Drosten C. Hosts and sources of endemic human coronaviruses. Adv Virus Res. 2018; 100: 163-88. [CrossRef]
- [85] Rashidzadeh H, Danafar H, Rahimi H, Mozafari F, Salehiabar M, Rahmati MA, et al. Nanotechnology against the novel coronavirus (severe acute respiratory syndrome coronavirus 2): diagnosis, treatment, therapy and future perspectives. Nanomedicine. 2021; 16(6): 497-516. [CrossRef]
- [86] Rahimi H, Salehiabar M, Barsbay M, Ghaffarlou M, Kavetskyy T, Sharafi A, et al. CRISPR systems for COVID-19 diagnosis. ACS Sens. 2021; 6(4): 1430-45. [CrossRef]
- [87] Su S, Wong G, Shi W, Liu J, Lai AC, Zhou J, et al. Epidemiology, genetic recombination, and pathogenesis of coronaviruses. Trends Microbiol. 2016; 24(6): 490-502. [CrossRef]
- [88] Zhang N-N, Li X-F, Deng Y-Q, Zhao H, Huang Y-J, Yang G, et al. A thermostable mRNA vaccine against COVID- 19. Cell. 2020; 182(5): 1271-83. [CrossRef]
- [89] Ge X-Y, Li J-L, Yang X-L, Chmura AA, Zhu G, Epstein JH, et al. Isolation and characterization of a bat SARS-like coronavirus that uses the ACE2 receptor. Nature. 2013; 503(7477): 535-8. [CrossRef]
- [90] Hoffmann M, Kleine-Weber H, Schroeder S, Krüger N, Herrler T, Erichsen S, et al. SARS-CoV-2 cell entry depends on ACE2 and TMPRSS2 and is blocked by a clinically proven protease inhibitor. Cell. 2020; 181(2): 271-80. [CrossRef]
- [91] Neuman BW, Kiss G, Kunding AH, Bhella D, Baksh MF, Connelly S, et al. A structural analysis of M protein in coronavirus assembly and morphology. J Struct Biol. 2011; 174(1): 11-22. [CrossRef]
- [92] Schoeman D, Fielding BC. Coronavirus envelope protein: current knowledge. Virol J. 2019; 16(1): 1-22. [CrossRef]
- [93] de Haan CA, Rottier PJ. Molecular interactions in the assembly of coronaviruses. Adv Virus Res. 2005; 64: 165-230. [CrossRef]
- [94] Matheson NJ, Lehner PJ. How does SARS-CoV-2 cause COVID-19? Science. 2020; 369(6503): 510-1. [CrossRef] [95] Pillay TS. Gene of the month: the 2019-nCoV/SARS-CoV-2 novel coronavirus spike protein. J Clin Pathol. 2020; 73(7): 366-9. [CrossRef]
- [96] Lima TLC, Feitosa RdC, Santos-Silva D, Santos-Silva D, Maria A, Siqueira EMdS, et al. Improving encapsulation of hydrophilic chloroquine diphosphate into biodegradable nanoparticles: a promising approach against herpes virus simplex-1 infection. Pharmaceutics. 2018; 10(4): 255. [CrossRef]
- [97] Huang Q, Ji K, Tian S, Wang F, Huang B, Tong Z, et al. A single-dose mRNA vaccine provides a long-term protection for hACE2 transgenic mice from SARS-CoV-2. Nat Commun. 2021; 12(1): 1-10. [CrossRef]
- [98] Krammer F. SARS-CoV-2 vaccines in development. Nature. 2020; 586(7830): 516-27. [CrossRef]
- [99] Elia U, Ramishetti S, Rosenfeld R, Dammes N, Bar-Haim E, Naidu GS, et al. Design of SARS-CoV-2 hFc-conjugated receptor-binding domain mRNA vaccine delivered via lipid nanoparticles. ACS Nano. 2021; 15(6): 9627-37. [CrossRef]
- [100] Elia U, Rotem S, Bar-Haim E, Ramishetti S, Naidu GS, Gur D, et al. Lipid nanoparticle RBD-hFc mRNA vaccine protects hACE2 transgenic mice against a lethal SARS-CoV-2 infection. Nano Lett. 2021; 21(11): 4774-9. [CrossRef]
- [101] de Alwis R, Gan ES, Chen S, Leong YS, Tan HC, Zhang SL, et al. A single dose of self-transcribing and replicating RNA-based SARS-CoV-2 vaccine produces protective adaptive immunity in mice. Mol Ther. 2021; 29(6): 1970-83. [CrossRef]
- [102] Park JW, Lagniton PN, Liu Y, Xu R-H. mRNA vaccines for COVID-19: what, why and how. Int J Biol Sci. 2021; 17(6): 1446. [CrossRef]
- [103] Rauch S, Roth N, Schwendt K, Fotin-Mleczek M, Mueller SO, Petsch B. mRNA-based SARS-CoV-2 vaccine candidate CVnCoV induces high levels of virus-neutralising antibodies and mediates protection in rodents. NPJ Vaccines. 2021; 6(1): 1-9. [CrossRef]
- [104] Huang J, Tao G, Liu J, Cai J, Huang Z, Chen J-x. Current prevention of COVID-19: natural products and herbal medicine. Front Pharmacol. 2020; 1635. [CrossRef]
- [105] Lu J, Lu G, Tan S, Xia J, Xiong H, Yu X, et al. A COVID-19 mRNA vaccine encoding SARS-CoV-2 virus-like particles induces a strong antiviral-like immune response in mice. Cell Res. 2020; 30(10): 936-9. [CrossRef]
- [106] McKay PF, Hu K, Blakney AK, Samnuan K, Brown JC, Penn R, et al. Self-amplifying RNA SARS-CoV-2 lipid nanoparticle vaccine candidate induces high neutralizing antibody titers in mice. Nat Commun. 2020; 11(1): 1-7. [CrossRef]
- [107] Lou G, Anderluzzi G, Schmidt ST, Woods S, Gallorini S, Brazzoli M, et al. Delivery of self-amplifying mRNA vaccines by cationic lipid nanoparticles: The impact of cationic lipid selection. J Control Release. 2020; 325: 370-9. [CrossRef]
- [108] Huang Q, Zeng J, Yan J. COVID-19 mRNA vaccines. J Genet Genomics. 2021; 48(2): 107-14. [CrossRef]
APA | WALWEEL N, KUTLU A, YILMAZ U, AL-SHAEBI Z, CHARMI J, AYDIN Ö (2022). Review: mRNA delivery systems in vaccinations and COVID-19. , 1084 - 1101. 10.29228/jrp.205 |
Chicago | WALWEEL Nada,KUTLU Aybuke Ulku,YILMAZ Ummugulsum,AL-SHAEBI Zakarya,CHARMI Jalil,AYDIN Ömer Review: mRNA delivery systems in vaccinations and COVID-19. (2022): 1084 - 1101. 10.29228/jrp.205 |
MLA | WALWEEL Nada,KUTLU Aybuke Ulku,YILMAZ Ummugulsum,AL-SHAEBI Zakarya,CHARMI Jalil,AYDIN Ömer Review: mRNA delivery systems in vaccinations and COVID-19. , 2022, ss.1084 - 1101. 10.29228/jrp.205 |
AMA | WALWEEL N,KUTLU A,YILMAZ U,AL-SHAEBI Z,CHARMI J,AYDIN Ö Review: mRNA delivery systems in vaccinations and COVID-19. . 2022; 1084 - 1101. 10.29228/jrp.205 |
Vancouver | WALWEEL N,KUTLU A,YILMAZ U,AL-SHAEBI Z,CHARMI J,AYDIN Ö Review: mRNA delivery systems in vaccinations and COVID-19. . 2022; 1084 - 1101. 10.29228/jrp.205 |
IEEE | WALWEEL N,KUTLU A,YILMAZ U,AL-SHAEBI Z,CHARMI J,AYDIN Ö "Review: mRNA delivery systems in vaccinations and COVID-19." , ss.1084 - 1101, 2022. 10.29228/jrp.205 |
ISNAD | WALWEEL, Nada vd. "Review: mRNA delivery systems in vaccinations and COVID-19". (2022), 1084-1101. https://doi.org/10.29228/jrp.205 |
APA | WALWEEL N, KUTLU A, YILMAZ U, AL-SHAEBI Z, CHARMI J, AYDIN Ö (2022). Review: mRNA delivery systems in vaccinations and COVID-19. Journal of research in pharmacy (online), 26(5), 1084 - 1101. 10.29228/jrp.205 |
Chicago | WALWEEL Nada,KUTLU Aybuke Ulku,YILMAZ Ummugulsum,AL-SHAEBI Zakarya,CHARMI Jalil,AYDIN Ömer Review: mRNA delivery systems in vaccinations and COVID-19. Journal of research in pharmacy (online) 26, no.5 (2022): 1084 - 1101. 10.29228/jrp.205 |
MLA | WALWEEL Nada,KUTLU Aybuke Ulku,YILMAZ Ummugulsum,AL-SHAEBI Zakarya,CHARMI Jalil,AYDIN Ömer Review: mRNA delivery systems in vaccinations and COVID-19. Journal of research in pharmacy (online), vol.26, no.5, 2022, ss.1084 - 1101. 10.29228/jrp.205 |
AMA | WALWEEL N,KUTLU A,YILMAZ U,AL-SHAEBI Z,CHARMI J,AYDIN Ö Review: mRNA delivery systems in vaccinations and COVID-19. Journal of research in pharmacy (online). 2022; 26(5): 1084 - 1101. 10.29228/jrp.205 |
Vancouver | WALWEEL N,KUTLU A,YILMAZ U,AL-SHAEBI Z,CHARMI J,AYDIN Ö Review: mRNA delivery systems in vaccinations and COVID-19. Journal of research in pharmacy (online). 2022; 26(5): 1084 - 1101. 10.29228/jrp.205 |
IEEE | WALWEEL N,KUTLU A,YILMAZ U,AL-SHAEBI Z,CHARMI J,AYDIN Ö "Review: mRNA delivery systems in vaccinations and COVID-19." Journal of research in pharmacy (online), 26, ss.1084 - 1101, 2022. 10.29228/jrp.205 |
ISNAD | WALWEEL, Nada vd. "Review: mRNA delivery systems in vaccinations and COVID-19". Journal of research in pharmacy (online) 26/5 (2022), 1084-1101. https://doi.org/10.29228/jrp.205 |