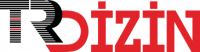
Determination of miR-373 and miR-204 levels in neuronal exosomes in Alzheimer’s disease
Yıl: 2022 Cilt: 52 Sayı: 5 Sayfa Aralığı: 1458 - 1467 Metin Dili: İngilizce DOI: 10.55730/1300-0144.5484 İndeks Tarihi: 27-12-2022
Determination of miR-373 and miR-204 levels in neuronal exosomes in Alzheimer’s disease
Öz: Background/aim: NLRP3 inflammasome activation has been known to be involved in the etiology and progression of Alzheimer’s disease (AD). Furthermore, AD and diabetes mellitus have common pathomechanisms. It has been shown that P2X7R whose expression is increased in brain tissues with AD and plays a role in the activation of NLRP3 inflammasome is suppressed by miR-373 in patients with osteoarthritis. Therefore, the question of whether the suppressive effect of miR-373 on NLRP3 may have a role in the pathophysiology of AD comes to mind. On the other hand, it is known that the miR-204 level increases in response to TXNIP, another NLRP3 inflammasome inducer with high expression in AD. In primary human islets, miR-204 reduces the expression of GLP-1R. It has been discovered that in vivo deletion of miR-204 is protective against diabetes by increasing GLP-1R and insulin secretion. Considering the relationship between miR-204 and TXNIP and the relationship of miR-204 with diabetes suggests investigating the effect of miR-204 on the inflammatory pathway in AD. Based on the common pathophysiological mechanisms between AD and diabetes and the reported changes related to NLRP3 inflammasome, we analyzed miR-373 and miR-204 in neuron-derived serum exosomes in this study. Neuron- derived exosomes in neurodegenerative diseases are considered to be better candidates for developing potential biomarkers. Materials and methods: The expression levels of miR-204 and miR-373 were investigated in neuron-derived serum exosomes obtained from 15 patients with mild AD, 18 with moderate AD, and 21 cognitively healthy individuals. Results and discussion: The miR-204 and miR-373 expressions were significantly decreased in both patient groups compared to the control group. Therefore, we suggest that miR-204 and miR-373 are potential biomarkers for AD. However, due to the preliminary nature of this study, further large-scale studies are needed to support our findings.
Anahtar Kelime: Belge Türü: Makale Makale Türü: Araştırma Makalesi Erişim Türü: Erişime Açık
- 1. Prince M, Wimo A, Guerchet M, Ali G, Wu Y et al. The global impact of dementia. World Alzheimer Report 2015; 1-82.
- 2. Mandrekar-Colucci S and Landreth GE. Microglia and inflammation in Alzheimer’s disease. CNS & Neurological Disorders-Drug Targets (Formerly Current Drug Targets-CNS & Neurological Disorders) 2010; 9 (2): 156-167. https://doi. org/10.2174/187152710791012071
- 3. Hebert LE, Weuve J, Scherr PA, and Evans DA. Alzheimer disease in the United States (2010–2050) estimated using the 2010 census. Neurology 2013; 80 (19): 1778-1783. https://doi. org/ 10.1212/WNL.0b013e31828726f5
- 4. Naj AC, Schellenberg GD, and Consortium AsDG. Genomic variants, genes, and pathways of Alzheimer’s disease: an overview. American Journal of Medical Genetics Part B: Neuropsychiatric Genetics 2017; 174 (1): 5-26. https://doi. org/10.1002/ajmg.b.32499
- 5. Mahley RW. Apolipoprotein E: cholesterol transport protein with expanding role in cell biology. Science 1988; 240 (4852): 622-630. https://doi.org/10.1126/science.3283935
- 6. Wightman DP, Jansen IE, Savage JE, Shadrin AA, Bahrami S et al. A genome-wide association study with 1,126,563 individuals identifies new risk loci for Alzheimer’s disease. Nature genetics 2021; 53 (9): 1276-1282. https://doi.org/10.1038/s41588-021- 00921-z
- 7. Shao W, Peng D, and Wang X. Genetics of Alzheimer’s disease: From pathogenesis to clinical usage. Journal of Clinical Neuroscience 2017; 45 1-8. https://doi.org/10.1016/j. jocn.2017.06.074
- 8. Wu Z-C, Yu J-T, Li Y, and Tan L. Clusterin in Alzheimer’s disease. Advances in clinical chemistry 2012; 56:155-73. https://doi.org/10.1016/b978-0-12-394317-0.00011-x
- 9. Harold D, Abraham R, Hollingworth P, Sims R, Gerrish A et al. Genome-wide association study identifies variants at CLU and PICALM associated with Alzheimer’s disease. Nature genetics 2009; 41 (10): 1088. https://doi.org/10.1038/ng.440
- 10. Jin C, Liu X, Zhang F, Wu Y, Yuan J et al. An updated meta- analysis of the association between SORL1 variants and the risk for sporadic Alzheimer’s disease. Journal of Alzheimer’s Disease 2013; 37 (2): 429-437. https://doi.org/10.3233/JAD- 130533
- 11. Chen H, Wu G, Jiang Y, Feng R, Liao M et al. Analyzing 54,936 samples supports the association between CD2AP rs9349407 polymorphism and Alzheimer’s disease susceptibility. Molecular neurobiology 2015; 52 (1): 1-7. https://doi. org/10.1007/s12035-014-8834-2
- 12. Wang Z, Lei H, Zheng M, Li Y, Cui Y et al. Meta-analysis of the association between Alzheimer disease and variants in GAB2, PICALM, and SORL1. Molecular neurobiology 2016; 53 (9): 6501-6510. https://doi.org/10.1007/s12035-015-9546-y
- 13. Cruchaga C, Karch CM, Jin SC, Benitez BA, Cai Y et al. Rare coding variants in the phospholipase D3 gene confer risk for Alzheimer’s disease. Nature 2014; 505 (7484): 550- 554. https:// doi.org/10.1038/nature12825
- 14. Wetzel-Smith MK, Hunkapiller J, Bhangale TR, Srinivasan K, Maloney JA et al. A rare mutation in UNC5C predisposes to late-onset Alzheimer’s disease and increases neuronal cell death. Nature medicine 2014; 20 (12): 1452-1457. https://doi. org/10.1038/nm.3736
- 15. Sanabria-Castro A, Alvarado-Echeverría I, and Monge-Bonilla C. Molecular pathogenesis of Alzheimer’s disease: an update. Annals of neurosciences 2017; 24 (1): 46-54. https://doi. org/10.1159/000464422
- 16. Heneka MT, Carson MJ, El Khoury J, Landreth GE, Brosseron F et al. Neuroinflammation in Alzheimer’s disease. The Lancet Neurology 2015; 14 (4): 388-405. https://doi.org/10.1016/ S1474- 4422(15)70016-5
- 17. Wang M, Qin L, and Tang B. MicroRNAs in Alzheimer’s disease. Frontiers in genetics 2019; 10:153. https://doi. org/10.3389/fgene.2019.00153
- 18. Janas AM, Sapoń K, Janas T, Stowell MH, and Janas T. Exosomes and other extracellular vesicles in neural cells and neurodegenerative diseases. Biochimica et Biophysica Acta (BBA)- Biomembranes 2016; 1858 (6): 1139-1151. https://doi. org/10.1016/j.bbamem.2016.02.011
- 19. Kanninen KM, Bister N, Koistinaho J, and Malm T. Exosomes as new diagnostic tools in CNS diseases. Biochimica et Biophysica Acta (BBA)-Molecular Basis of Disease 2016; 1862 (3): 403-410. https://doi.org/10.1016/j. bbadis.2015.09.020
- 20. Halle A, Hornung V, Petzold GC, Stewart CR, Monks BG et al. The NALP3 inflammasome is involved in the innate immune response to amyloid-β. Nature immunology 2008; 9 (8): 857. https://doi.org/10.1038/ni.1636
- 21. Parvathenani LK, Tertyshnikova S, Greco CR, Roberts SB, Robertson B et al. P2X7 mediates superoxide production in primary microglia and is up-regulated in a transgenic mouse model of Alzheimer’s disease. Journal of Biological Chemistry 2003; 278 (15): 13309-13317. https://doi.org/10.1074/jbc. M209478200
- 22. Zhang W, Zhong B, Zhang C, Luo C, and Zhan Y. miR-373 regulates inflammatory cytokine- mediated chondrocyte proliferation in osteoarthritis by targeting the P2X7 receptor. FEBS open bio 2018; 8 (3): 325-331. https://doi. org/10.1002/2211-5463.12345
- 23. Sørensen SS, Nygaard A-B, and Christensen T. miRNA expression profiles in cerebrospinal fluid and blood of patients with Alzheimer’s disease and other types of dementia–an exploratory study. Translational neurodegeneration 2016; 5 (1): 6. https://doi.org/10.1186/ s40035-016- 0053-5
- 24. Zhou R, Tardivel A, Thorens B, Choi I, and Tschopp J. Thioredoxin-interacting protein links oxidative stress to inflammasome activation. Nature immunology 2010; 11 (2): 136-140. https://doi.org/ 10.1038/ni.1831
- 25. Su CJ, Feng Y, Liu TT, Liu X, Bao JJ et al. Thioredoxin interacting protein induced α synuclein accumulation via inhibition of autophagic flux: Implications for Parkinson’s disease. CNS neuroscience & therapeutics 2017; 23 (9): 717- 723. https://doi.org/10.1111/cns.12721
- 26. Li L, Ismael S, Nasoohi S, Sakata K, Liao F-F et al. Thioredoxin- interacting protein (TXNIP) associated NLRP3 inflammasome activation in human Alzheimer’s disease brain. Journal of Alzheimer’s Disease 2019; 68 (1): 255-265. https://doi. org/10.3233/JAD-180814
- 27. Melone MAB, Dato C, Paladino S, Coppola C, Trebini C et al. Verapamil inhibits Ser202/Thr205 phosphorylation of tau by blocking TXNIP/ROS/p38 MAPK pathway. Pharmaceutical research 2018; 35 (2): 1-14. https://doi.org/10.1007/s11095- 017-2276-2
- 28. Jo S, Chen J, Xu G, Grayson TB, Thielen LA et al. miR-204 controls glucagon-like peptide 1 receptor expression and agonist function. Diabetes 2018; 67 (2): 256-264. https://doi. org/10.2337/db17-0506
- 29. Xu W, von Strauss E, Qiu C, Winblad B, and Fratiglioni L. Uncontrolled diabetes increases the risk of Alzheimer’s disease: a population-based cohort study. Diabetologia 2009; 52 (6): 1031. https://doi.org/10.1007/s00125-009-1323-x
- 30. Femminella GD and Edison P. Evaluation of neuroprotective effect of glucagon-like peptide 1 analogs using neuroimaging. Alzheimer’s & Dementia 2014; 10: S55-S61. https://doi. org/10.1016/j.jalz.2013.12.012
- 31. Perry T and Greig NH. Enhancing central nervous system endogenous GLP-1 receptor pathways for intervention in Alzheimer’s disease. Current Alzheimer Research 2005; 2 (3): 377-385. https://doi.org/10.2174/1567205054367892
- 32. Liang C, Zhu H, Xu Y, Huang L, Ma C et al. MicroRNA-153 negatively regulates the expression of amyloid precursor protein and amyloid precursor-like protein 2 . Brain research 2012; 1455:103-13. https://doi.org/10.1016/j.brainres.2011.10.051
- 33. Tiribuzi R, Crispoltoni L, Porcellati S, Di Lullo M, Florenzano F et al. miR128 up-regulation correlates with impaired amyloid β (1-42) degradation in monocytes from patients with sporadic Alzheimer’s disease. Neurobiology of aging 2014; 35 (2): 345- 356. https://doi.org/ 10.1016/j.neurobiolaging.2013.08.003
- 34. Lee K, Kim H, An K, Kwon O-B, Park S et al. Replenishment of microRNA-188-5p restores the synaptic and cognitive deficits in 5XFAD Mouse Model of Alzheimer’s Disease. Scientific reports 2016; 6 (1): 1-14. https://doi.org/10.1038/srep34433
- 35. Wang X, Tan L, Lu Y, Peng J, Zhu Y et al. MicroRNA-138 promotes tau phosphorylation by targeting retinoic acid receptor alpha. FEBS letters 2015; 589 (6): 726-729. https://doi. org/10.1016/j.febslet.2015.02.001
- 36. He D, Tan J, and Zhang J. miR-137 attenuates Aβ-induced neurotoxicity through inactivation of NF-κB pathway by targeting TNFAIP1 in Neuro2a cells. Biochemical and biophysical research communications 2017; 490 (3): 941-947. https://doi.org/10.1016/j.bbrc.2017.06.144
- 37. Souza VC, Morais Jr GS, Henriques AD, Machado-Silva W, Perez DIV et al. Whole-blood levels of MicroRNA-9 are decreased in patients with late-onset Alzheimer disease. American Journal of Alzheimer’s Disease & Other Dementias® 2020; 35:1533317520911573. https://doi.org/ 10.1177/1533317520911573
- 38. Giuliani A, Gaetani S, Sorgentoni G, Agarbati S, Laggetta M et al. Circulating Inflamma-miRs as Potential Biomarkers of Cognitive Impairment in Patients Affected by Alzheimer’s Disease. Frontiers in Aging Neuroscience 2021; 13: 647015. https://doi.org/10.3389/fnagi.2021.647015
- 39. McKhann GM, Knopman DS, Chertkow H, Hyman BT, Jack Jr CR et al. The diagnosis of dementia due to Alzheimer’s disease: recommendations from the National Institute on Aging- Alzheimer’s Association workgroups on diagnostic guidelines for Alzheimer’s disease. Alzheimer’s & dementia 2011; 7 (3): 263-269. https://doi.org/10.1016/j.jalz.2011.03.005
- 40. Morris JC. Clinical dementia rating: a reliable and valid diagnostic and staging measure for dementia of the Alzheimer type. International psychogeriatrics 1997; 9 (S1): 173-176. https://doi.org/ 10.1017/s1041610297004870
- 41. Scheltens P, Launer LJ, Barkhof F, Weinstein HC, and van Gool WA. Visual assessment of medial temporal lobe atrophy on magnetic resonance imaging: interobserver reliability. Journal of neurology 1995; 242 (9): 557-560. https://doi.org/10.1007/ BF00868807
- 42. Scheltens P, Erkinjunti T, Leys D, Wahlund L-O, Inzitari D et al. White matter changes on CT and MRI: an overview of visual rating scales. European neurology 1998; 39 (2): 80-89. https:// doi.org/10.1159/000007921
- 43. Folstein MF, Folstein SE, and McHugh PR. “Mini-mental state”: a practical method for grading the cognitive state of patients for the clinician. Journal of psychiatric research 1975; 12 (3): 189-198. https://doi.org/10.1016/0022-3956(75)90026-6
- 44. Li M, Rai AJ, DeCastro GJ, Zeringer E, Barta T et al. An optimized procedure for exosome isolation and analysis using serum samples: application to cancer biomarker discovery. Methods 2015; 87:26-30. https://doi.org/10.1016/j. ymeth.2015.03.009
- 45. Mustapic M, Eitan E, Werner Jr JK, Berkowitz ST, Lazaropoulos MP et al. Plasma extracellular vesicles enriched for neuronal origin: a potential window into brain pathologic processes. Frontiers in neuroscience 2017; 11: 278. https://doi. org/10.3389/fnins.2017.00278
- 46. Livak KJ and Schmittgen TD. Analysis of relative gene expression data using real-time quantitative PCR and the 2− ΔΔCT method. methods 2001; 25 (4): 402-408. https://doi. org/10.1006/meth.2001.1262
- 47. Thompson AG, Gray E, Heman-Ackah SM, Mäger I, Talbot K et al. Extracellular vesicles in neurodegenerative disease— pathogenesis to biomarkers. Nature Reviews Neurology 2016; 12 (6): 346-357. https://doi.org/10.1038/nrneurol.2016.68
- 48. Jain G, Stuendl A, Rao P, Berulava T, Centeno TP et al. A combined miRNA–piRNA signature to detect Alzheimer’s disease. Translational psychiatry 2019; 9 (1): 1-12. https://doi. org/10.1038/s41398-019-0579-2
- 49. Goetzl EJ, Boxer A, Schwartz JB, Abner EL, Petersen RC et al. Altered lysosomal proteins in neural-derived plasma exosomes in preclinical Alzheimer disease. Neurology 2015; 85 (1): 40- 47. https://doi.org/10.1212/WNL.0000000000001702
- 50. Walsh DM, Cha DJ, Mengel D, Mustapic M, Liu W et al. miR-212 and miR-132 are down- regulated in neurally-derived plasma exosomes of Alzheimer’s patients. Frontiers in neuroscience 2019; 13: 1208. https://doi.org/10.3389/fnins.2019.01208
- 51. Serpente M, Fenoglio C, D’Anca M, Arcaro M, Sorrentino F et al. MiRNA Profiling in Plasma Neural-Derived Small Extracellular Vesicles from Patients with Alzheimer’s Disease. Cells 2020; 9 (6): 1443. https://doi.org/10.3390/cells9061443
- 52. Hornung S, Dutta S, and Bitan G. CNS-Derived Blood Exosomes as a Promising Source of Biomarkers: Opportunities and Challenges. Frontiers in Molecular Neuroscience 2020; 13: 38. https://doi.org/10.3389/ fnmol.2020.00038
- 53. Schneider R, McKeever P, Kim T, Graff C, Van Swieten JC et al. Downregulation of exosomal miR-204-5p and miR-632 as a biomarker for FTD: a GENFI study. Journal of Neurology, Neurosurgery & Psychiatry 2018; 89 (8): 851-858. https://doi. org/10.1136/jnnp-2017-317492
- 54. Inohara N, Ding L, Chen S, and Núñez G. harakiri, a novel regulator of cell death, encodes a protein that activates apoptosis and interacts selectively with survival-promoting proteins Bcl-2 and Bcl-XL. The EMBO journal 1997; 16 (7): 1686-1694. https://doi.org/10.1093/emboj/16.7.1686
- 55. Zhu X, Yu L, Tao W, Jin J, and Xu Y. O3-01-05: miR-204 attenuates memory deficits in a mouse model of Alzheimer’s disease. Alzheimer’s & Dementia 2019; 15: P876-P877.
- 56. Olsson B, Lautner R, Andreasson U, Öhrfelt A, Portelius E et al. CSF and blood biomarkers for the diagnosis of Alzheimer’s disease: a systematic review and meta-analysis. The Lancet Neurology 2016; 15 (7): 673-684. https://doi.org/10.1016/ S1474-4422(16)00070-3
- 57. Jack Jr CR, Bennett DA, Blennow K, Carrillo MC, Dunn B et al. NIA-AA research framework: toward a biological definition of Alzheimer’s disease. Alzheimer’s & Dementia 2018; 14 (4): 535-562. https://doi.org/10.1016/j.jalz.2018.02.018
- 58. Janelidze S, Stomrud E, Smith R, Palmqvist S, Mattsson N et al. Cerebrospinal fluid p-tau217 performs better than p-tau181 as a biomarker of Alzheimer’s disease. Nature communications 2020; 11 (1): 1-12. https://doi.org/10.1038/s41467-020-15436- 0
- 59. Janelidze S, Berron D, Smith R, Strandberg O, Proctor NK et al. Associations of Plasma Phospho-Tau217 Levels With Tau Positron Emission Tomography in Early Alzheimer Disease. JAMA neurology 2020; 78(2): 149-156. https://doi. org/10.1001/jamaneurol.2020.4201
APA | Taşdelen E, Ozel-Kizil E, Tezcan Aydemir S, Yalap Ö, Bingöl A, KUTLAY N (2022). Determination of miR-373 and miR-204 levels in neuronal exosomes in Alzheimer’s disease. , 1458 - 1467. 10.55730/1300-0144.5484 |
Chicago | Taşdelen Elifcan,Ozel-Kizil Erguvan Tugba,Tezcan Aydemir Sabiha,Yalap Ömer Eray,Bingöl Ayşe Petek,KUTLAY NÜKET Determination of miR-373 and miR-204 levels in neuronal exosomes in Alzheimer’s disease. (2022): 1458 - 1467. 10.55730/1300-0144.5484 |
MLA | Taşdelen Elifcan,Ozel-Kizil Erguvan Tugba,Tezcan Aydemir Sabiha,Yalap Ömer Eray,Bingöl Ayşe Petek,KUTLAY NÜKET Determination of miR-373 and miR-204 levels in neuronal exosomes in Alzheimer’s disease. , 2022, ss.1458 - 1467. 10.55730/1300-0144.5484 |
AMA | Taşdelen E,Ozel-Kizil E,Tezcan Aydemir S,Yalap Ö,Bingöl A,KUTLAY N Determination of miR-373 and miR-204 levels in neuronal exosomes in Alzheimer’s disease. . 2022; 1458 - 1467. 10.55730/1300-0144.5484 |
Vancouver | Taşdelen E,Ozel-Kizil E,Tezcan Aydemir S,Yalap Ö,Bingöl A,KUTLAY N Determination of miR-373 and miR-204 levels in neuronal exosomes in Alzheimer’s disease. . 2022; 1458 - 1467. 10.55730/1300-0144.5484 |
IEEE | Taşdelen E,Ozel-Kizil E,Tezcan Aydemir S,Yalap Ö,Bingöl A,KUTLAY N "Determination of miR-373 and miR-204 levels in neuronal exosomes in Alzheimer’s disease." , ss.1458 - 1467, 2022. 10.55730/1300-0144.5484 |
ISNAD | Taşdelen, Elifcan vd. "Determination of miR-373 and miR-204 levels in neuronal exosomes in Alzheimer’s disease". (2022), 1458-1467. https://doi.org/10.55730/1300-0144.5484 |
APA | Taşdelen E, Ozel-Kizil E, Tezcan Aydemir S, Yalap Ö, Bingöl A, KUTLAY N (2022). Determination of miR-373 and miR-204 levels in neuronal exosomes in Alzheimer’s disease. Turkish Journal of Medical Sciences, 52(5), 1458 - 1467. 10.55730/1300-0144.5484 |
Chicago | Taşdelen Elifcan,Ozel-Kizil Erguvan Tugba,Tezcan Aydemir Sabiha,Yalap Ömer Eray,Bingöl Ayşe Petek,KUTLAY NÜKET Determination of miR-373 and miR-204 levels in neuronal exosomes in Alzheimer’s disease. Turkish Journal of Medical Sciences 52, no.5 (2022): 1458 - 1467. 10.55730/1300-0144.5484 |
MLA | Taşdelen Elifcan,Ozel-Kizil Erguvan Tugba,Tezcan Aydemir Sabiha,Yalap Ömer Eray,Bingöl Ayşe Petek,KUTLAY NÜKET Determination of miR-373 and miR-204 levels in neuronal exosomes in Alzheimer’s disease. Turkish Journal of Medical Sciences, vol.52, no.5, 2022, ss.1458 - 1467. 10.55730/1300-0144.5484 |
AMA | Taşdelen E,Ozel-Kizil E,Tezcan Aydemir S,Yalap Ö,Bingöl A,KUTLAY N Determination of miR-373 and miR-204 levels in neuronal exosomes in Alzheimer’s disease. Turkish Journal of Medical Sciences. 2022; 52(5): 1458 - 1467. 10.55730/1300-0144.5484 |
Vancouver | Taşdelen E,Ozel-Kizil E,Tezcan Aydemir S,Yalap Ö,Bingöl A,KUTLAY N Determination of miR-373 and miR-204 levels in neuronal exosomes in Alzheimer’s disease. Turkish Journal of Medical Sciences. 2022; 52(5): 1458 - 1467. 10.55730/1300-0144.5484 |
IEEE | Taşdelen E,Ozel-Kizil E,Tezcan Aydemir S,Yalap Ö,Bingöl A,KUTLAY N "Determination of miR-373 and miR-204 levels in neuronal exosomes in Alzheimer’s disease." Turkish Journal of Medical Sciences, 52, ss.1458 - 1467, 2022. 10.55730/1300-0144.5484 |
ISNAD | Taşdelen, Elifcan vd. "Determination of miR-373 and miR-204 levels in neuronal exosomes in Alzheimer’s disease". Turkish Journal of Medical Sciences 52/5 (2022), 1458-1467. https://doi.org/10.55730/1300-0144.5484 |