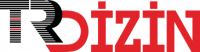
Effect of microfluidic channel integration onto gold microelectrode on its redox electrochemistry
Yıl: 2023 Cilt: 47 Sayı: 1 Sayfa Aralığı: 232 - 241 Metin Dili: İngilizce DOI: 10.55730/1300-0527.3532 İndeks Tarihi: 13-03-2023
Effect of microfluidic channel integration onto gold microelectrode on its redox electrochemistry
Öz: Microfluidic systems have attracted significant interest in recent years as they are extensively employed in lab-on-chip and organ-on-chip research. Their combination with electrochemical platforms offers many advantages, promising a high potential for sensing applications, still the microfluidic-channel integration onto electrodes might induce challenges related to changes in signal-to- noise ratios and mass transport conditions. In this study, we investigated the effect of microfluidic channel integration in redox behavior of thermally deposited gold thin film microelectrodes by voltammetric (CV and SWV) electrochemical measurements. Using different dimensions of PDMS microfluidic channels (i.e. widths of 50, 100, 250, and 500 μm) and a constant electrode dimension (200 μm), we analyzed the relationship between altered electroactive area and electrochemical response against target redox molecules. The increases in electroactive area which were determined by the microfluidic channel sizes were in well-correlation with the obtained CV and SWV redox currents as expected. There was no significant decrease in signal-to-noise ratio in microchannel-integrated electrodes. AFM and SEM characterization demonstrated that thermally deposited thin film electrodes had significantly lower (approximately 25 fold) surface roughness in comparison to commercial screen-printed electrodes. Additionally, we have observed a clear microelectrode-to- macroelectrode transition, from hemispherical to linear (planar) diffusion in other terms, with the increasing channel size.
Anahtar Kelime: Belge Türü: Makale Makale Türü: Araştırma Makalesi Erişim Türü: Erişime Açık
- 1. Guo L, Feng J, Fang Z, Xu J, Lu X. Application of microfluidic “lab-on-a-chip” for the detection of mycotoxins in foods. Trends in Food Science and Technology 2015; 46 (2): 252–263. https://doi.org/10.1016/j.tifs.2015.09.005
- 2. Pol R, Céspedes F, Gabriel D, Baeza M. Microfluidic lab-on-a-chip platforms for environmental monitoring. TrAC Trends in Analytical Chemistry 2017; 95: 62–68. https://doi.org/ 10.1016/j.trac.2017.08.001
- 3. Kaur J, Ghorbanpoor H, Öztürk Y, Kaygusuz Ö, Avcı H, Darcan C et al. On chip label free impedance based detection of antibiotic permeation. IET Nanobiotechnology 2021; 15 (1): 100–106. https://doi.org/10.1049/nbt2.12019
- 4. Bhatia SN, Ingber DE. Microfluidic organs-on-chips. Nature Biotechnology 2014; 32 (8): 760–772. https://doi.org/10.1038/nbt.2989
- 5. Bein A, Shin W, Jalili-Firoozinezhad S, Park MH, Sontheimer-Phelps A, Tovaglieri A et al. Microfluidic Organ-on-a-Chip Models of Human Intestine. Cellular and Molecular Gastroenterology and Hepatology 2018; 5 (4): 659–668. https://doi.org/10.1016/j.jcmgh.2017.12.010
- 6. Srinivasan V, Pamula VK, Fair RB. An integrated digital microfluidic lab-on-a-chip for clinical diagnostics on human physiological fluids. Lab on a Chip 2004; 4 (4): 310–315. https://doi.org/10.1039/B403341H
- 7. Jung W, Han J, Choi JW, Ahn CH. Point-of-care testing (POCT) diagnostic systems using microfluidic lab-on-a-chip technologies. Microelectronic Engineering 2015; 132: 46–57. https://doi.org/10.1016/j.mee.2014.09.024
- 8. Wang S, Hong S, Cai S, Lei J, Chen J, Zhang N et al. Negative depletion mediated brightfield circulating tumour cell identification strategy on microparticle-based microfluidic chip. Journal of Nanobiotechnology 2020; 18: 70. https://doi.org/10.1186/s12951-020-00623-4
- 9. Khamenehfar A, Beischlag T V., Russell PJ, Ling MTP, Nelson C, Li PCH. Label-free isolation of a prostate cancer cell among blood cells and the single-cell measurement of drug accumulation using an integrated microfluidic chip. Biomicrofluidics 2015; 9 (6): 064104. https:// doi.org/10.1063/1.4934715
- 10. Liao Z, Zhang Y, Li Y, Miao Y, Gao S, Lin F et al. Microfluidic chip coupled with optical biosensors for simultaneous detection of multiple analytes: A review. Biosensors and Bioelectronics 2019; 126: 697–706. https://doi.org/10.1016/j.bios.2018.11.032
- 11. Schmidt-Speicher LM, Länge K. Microfluidic integration for electrochemical biosensor applications. Current Opinion in Electrochemistry 2021; 29: 100755. https://doi.org/10.1016/j.coelec.2021.100755
- 12. Ucar A, González-Fernández E, Staderini M, Avlonitis N, Murray AF, Bradley M et al. Miniaturisation of a peptide-based electrochemical protease activity sensor using platinum microelectrodes. Analyst 2020; 145 (3): 975–982. https://doi.org/10.1039/C9AN02321F
- 13. Bard AJ, Faulkner LR, White HS. Electrochemical methods: fundamentals and applications. John Wiley & Sons, 2022
- 14. Amatore C, Pebay C, Thouin L, Wang A, Warkocz J-S. Difference between Ultramicroelectrodes and Microelectrodes: Influence of Natural Convection. Analytical Chemistry 2010; 82 (16): 6933–6939. https://doi.org/10.1021/ac101210r
- 15. Ronkainen NJ. Micro-and Nanoelectrodes in Protein-Based Electrochemical Biosensors for Nanomedicine and Other Applications. Advanced Bioelectronic Materials. Wiley, 2015
- 16. Cohen AE, Kunz RR. Large-area interdigitated array microelectrodes for electrochemical sensing. Sensors and Actuators B: Chemical 2000; 62 (1): 23–29. https://doi.org/10.1016/S0925-4005(99)00372-X
- 17. González-Fernández E, Staderini M, Marland JRK, Gray ME, Uçar A, Dunare C et al. In vivo application of an implantable tri- anchored methylene blue-based electrochemical pH sensor. Biosensors and Bioelectronics 2022; 197: 113728. https://doi.org/10.1016/j. bios.2021.113728
- 18. Quan Li P, Piper A, Schmueser I, Mount AR, Corrigan DK. Impedimetric measurement of DNA-DNA hybridisation using microelectrodes with different radii for detection of methicillin resistant: Staphylococcus aureus (MRSA). Analyst 2017; 142: 1946–1952. https://doi. org/10.1039/C7AN00436B
- 19. Huang XJ, O’Mahony AM, Compton RG. Microelectrode arrays for electrochemistry: Approaches to fabrication. Small 2009; 5 (7): 776– 788. https://doi.org/10.1002/smll.200801593
- 20. Shandhi MMH, Negi S. Fabrication of Out-of-Plane High Channel Density Microelectrode Neural Array with 3D Recording and Stimulation Capabilities. Journal of Microelectromechanical Systems 2020; 29 (4): 522–531. https://doi.org/10.1109/JMEMS.2020.3004847
- 21. Li X, Tian Y, Xia P, Luo Y, Rui Q. Fabrication of TiO2 and metal nanoparticle-microelectrode arrays by photolithography and site-selective photocatalytic deposition. Analytical Chemistry 2009; 81 (19): 8249–8255. https://doi.org/10.1021/ac9009879
- 22. Gomes VP, Pascon AM, Panepucci RR, Swart JW. Maskless production of neural-recording graphene microelectrode arrays. Journal of Vacuum Science & Technology B 2019; 37 (2): 022202. https://doi.org/10.1116/1.5048216
- 23. Yoo YK, Kim G, Park D, Kim J, Kim YS, Yun Kim H et al. Gold nanoparticles assisted sensitivity improvement of interdigitated microelectrodes biosensor for amyloid-β detection in plasma sample. Sensors and Actuators B: Chemical 2020; 308: 127710. https://doi. org/10.1016/j.snb.2020.127710
- 24. Geng Y, Zhu Z, Wang Y, Wang Y, Ouyang S, Zheng K et al. Multiplexing microelectrodes for dielectrophoretic manipulation and electrical impedance measurement of single particles and cells in a microfluidic device. Electrophoresis 2019; 40 (10): 1436–1445. https://doi. org/10.1002/elps.201800433
- 25. Ghorbanpoor H, Dizaji AN, Akcakoca I, Blair EO, Ozturk Y, Hoskisson P et al. A fully integrated rapid on-chip antibiotic susceptibility test – A case study for Mycobacterium smegmatis. Sensors and Actuators A: Physical 2022; 339: 113515. https://doi.org/10.1016/j. sna.2022.113515
- 26. Javidanbardan A, Chu V, Conde JP, Azevedo AM. Microchromatography integrated with impedance sensor for bioprocess optimization: Experimental and numerical study of column efficiency for evaluation of scalability. Journal of Chromatography A 2022; 1661: 462678. https://doi.org/10.1016/j.chroma.2021.462678
- 27. Song R, Xiong Q, Wu T, Ning X, Zhang F, Wang Q et al. Real-time monitoring of extracellular pH using a pH-potentiometric sensing SECM dual-microelectrode. Analytical and Bioanalytical Chemistry 2020; 412 (15): 3737–3743. https://doi.org/10.1007/s00216-020- 02625-5
- 28. Ghorbanpoor H, Corrigan D, Guzel FD. Effect of microchannel dimensions in electrochemical impedance spectroscopy using gold microelectrode. Sakarya University Journal of Science 2022; 26 (1): 120–127. https://doi.org/10.16984/saufenbilder.982707
- 29. Douglass EF, Driscoll PF, Liu D, Burnham NA, Lambert CR, McGimpsey WG. Effect of electrode roughness on the capacitive behavior of self-assembled monolayers. Analytical Chemistry 2008; 80 (20): 7670–7677. https://doi.org/10.1021/ac800521z
- 30. Li C, Zhang J, Han J, Yao B. A numerical solution to the effects of surface roughness on water–coal contact angle. Scientific Reports 2021; 11: 459. https://doi.org/10.1038/s41598-020-80729-9
- 31. Pearce TM, Wilson JA, Oakes SG, Chiu SY, Williams JC. Integrated microelectrode array and microfluidics for temperature clamp of sensory neurons in culture. Lab on a Chip 2005; 5: 97–101. https://doi.org/10.1039/B407871C
- 32. Goluch ED, Wolfrum B, Singh PS, Zevenbergen MAG, Lemay SG. Redox cycling in nanofluidic channels using interdigitated electrodes. Analytical and Bioanalytical Chemistry 2009; 394 (2): 447–456. https://doi.org/10.1007/s00216-008-2575-x
- 33. Mitchell P. Microfluidics—downsizing large-scale biology. Nature Biotechnology 2001; 19 (8): 717–721. https://doi.org/10.1038/90754
- 34. Compton RG, Banks CE. Understanding voltammetry. World Scientific, 2018
- 35. Zanello P, Nervi C, De Biani FF. Inorganic electrochemistry: theory, practice and application. Royal Society of Chemistry, 2019
- 36. Hussain G, Silvester DS. Comparison of Voltammetric Techniques for Ammonia Sensing in Ionic Liquids. Electroanalysis 2018; 30: 75–83. https://doi.org/10.1002/elan.201700555
- 37. Horny MC, Lazerges M, Siaugue JM, Pallandre A, Rose D, Bedioui F et al. Electrochemical DNA biosensors based on long-range electron transfer: Investigating the efficiency of a fluidic channel microelectrode compared to an ultramicroelectrode in a two-electrode setup. Lab on a Chip 2016; 16 (22): 4373–4381. https://doi.org/10.1039/C6LC00869K
- 38. Chen IJ, White IM. High-sensitivity electrochemical enzyme-linked assay on a microfluidic interdigitated microelectrode. Biosensors and Bioelectronics 2011; 26 (11): 4375–4381. https://doi.org/10.1016/j.bios.2011.04.044
- 39. Basheer EA, Bari HA. Study on the Effects of Electrode and Microchannel Sizes on the Performance of MBEB. Indian Journal of Science and Technology 2017; 10 (2): 1–4. https://doi.org/10.17485/ijst/2017/v10i(2)/110378
APA | Ucar A, Ali Z, Ghorbanpoor H, Didarian R, Doğan Güzel F (2023). Effect of microfluidic channel integration onto gold microelectrode on its redox electrochemistry. , 232 - 241. 10.55730/1300-0527.3532 |
Chicago | Ucar Ahmet,Ali Zahraa,Ghorbanpoor Hamed,Didarian Reza,Doğan Güzel Fatma Effect of microfluidic channel integration onto gold microelectrode on its redox electrochemistry. (2023): 232 - 241. 10.55730/1300-0527.3532 |
MLA | Ucar Ahmet,Ali Zahraa,Ghorbanpoor Hamed,Didarian Reza,Doğan Güzel Fatma Effect of microfluidic channel integration onto gold microelectrode on its redox electrochemistry. , 2023, ss.232 - 241. 10.55730/1300-0527.3532 |
AMA | Ucar A,Ali Z,Ghorbanpoor H,Didarian R,Doğan Güzel F Effect of microfluidic channel integration onto gold microelectrode on its redox electrochemistry. . 2023; 232 - 241. 10.55730/1300-0527.3532 |
Vancouver | Ucar A,Ali Z,Ghorbanpoor H,Didarian R,Doğan Güzel F Effect of microfluidic channel integration onto gold microelectrode on its redox electrochemistry. . 2023; 232 - 241. 10.55730/1300-0527.3532 |
IEEE | Ucar A,Ali Z,Ghorbanpoor H,Didarian R,Doğan Güzel F "Effect of microfluidic channel integration onto gold microelectrode on its redox electrochemistry." , ss.232 - 241, 2023. 10.55730/1300-0527.3532 |
ISNAD | Ucar, Ahmet vd. "Effect of microfluidic channel integration onto gold microelectrode on its redox electrochemistry". (2023), 232-241. https://doi.org/10.55730/1300-0527.3532 |
APA | Ucar A, Ali Z, Ghorbanpoor H, Didarian R, Doğan Güzel F (2023). Effect of microfluidic channel integration onto gold microelectrode on its redox electrochemistry. Turkish Journal of Chemistry, 47(1), 232 - 241. 10.55730/1300-0527.3532 |
Chicago | Ucar Ahmet,Ali Zahraa,Ghorbanpoor Hamed,Didarian Reza,Doğan Güzel Fatma Effect of microfluidic channel integration onto gold microelectrode on its redox electrochemistry. Turkish Journal of Chemistry 47, no.1 (2023): 232 - 241. 10.55730/1300-0527.3532 |
MLA | Ucar Ahmet,Ali Zahraa,Ghorbanpoor Hamed,Didarian Reza,Doğan Güzel Fatma Effect of microfluidic channel integration onto gold microelectrode on its redox electrochemistry. Turkish Journal of Chemistry, vol.47, no.1, 2023, ss.232 - 241. 10.55730/1300-0527.3532 |
AMA | Ucar A,Ali Z,Ghorbanpoor H,Didarian R,Doğan Güzel F Effect of microfluidic channel integration onto gold microelectrode on its redox electrochemistry. Turkish Journal of Chemistry. 2023; 47(1): 232 - 241. 10.55730/1300-0527.3532 |
Vancouver | Ucar A,Ali Z,Ghorbanpoor H,Didarian R,Doğan Güzel F Effect of microfluidic channel integration onto gold microelectrode on its redox electrochemistry. Turkish Journal of Chemistry. 2023; 47(1): 232 - 241. 10.55730/1300-0527.3532 |
IEEE | Ucar A,Ali Z,Ghorbanpoor H,Didarian R,Doğan Güzel F "Effect of microfluidic channel integration onto gold microelectrode on its redox electrochemistry." Turkish Journal of Chemistry, 47, ss.232 - 241, 2023. 10.55730/1300-0527.3532 |
ISNAD | Ucar, Ahmet vd. "Effect of microfluidic channel integration onto gold microelectrode on its redox electrochemistry". Turkish Journal of Chemistry 47/1 (2023), 232-241. https://doi.org/10.55730/1300-0527.3532 |