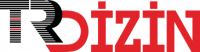
Bitki Peptit ve Amino Asit Taşıyıcılarının Demir Taşınmasındaki Görevleri
Yıl: 2022 Cilt: 10 Sayı: sp1 Sayfa Aralığı: 2646 - 2655 Metin Dili: Türkçe DOI: 10.24925/turjaf.v10isp1.2646-2655.5600 İndeks Tarihi: 22-05-2023
Bitki Peptit ve Amino Asit Taşıyıcılarının Demir Taşınmasındaki Görevleri
Öz: Demir (Fe) bitkiler için önemli bir mikro-besin elementi olup, eksikliği bitki büyümesi ve gelişimini engelleyerek ciddi verim kayıplarına neden olur. Toprakta bulunan yarayışlı demirin bitkiler tarafından alınması ve bitki organlarına taşınması üzerine uzun yıllara dayanan detaylı çalışmalar gerçekleştirilmiştir. Bu çalışmalar sonucunda demirin bazı organik maddeler ile şelatlanarak taşınabileceği gösterilmiştir. Bu organik maddelerin içerisinde peptitler ve amino asitler yer almaktadır. Ancak, demirin peptitler ve amino asitler ile bitki içerisine alımı veya organlar arası taşınması üzerine detaylı çalışmalar yapılmamıştır. Gerçekleştirilen az sayıdaki çalışmada oligopeptit taşıyıcı (OPT) ve İlaç/Metabolit Taşıyıcı (DMT) aileleri üzerine odaklanılmıştır. Yakın zaman önce bitkilerde keşfedilen UMAMIT amino asit taşıyıcı ailesinin demir taşınmasındaki olası rolleri ise henüz incelenmemiştir. Bu derleme kapsamında, demirin bitki içine alınması ve organlar arası taşınmasından sorumlu taşıyıcı aileleri ile OPT, DMT ve UMAMIT ailelerinin demir-peptit ve demir- amino asit komplekslerini taşımadaki rolleri özetlenmiştir.
Anahtar Kelime: The Roles of Plant Peptide and Amino Acid Transporters in Iron Transport
Öz: Iron (Fe) is an important micronutrient for plants, and its deficiency causes serious yield losses by inhibiting plant growth and development. Detailed studies have been carried out for many years on the uptake of available iron in the soil by plants and its transport to plant organs. These studies proved that Fe can be transported in chelated form with some organic substances including peptides and amino acids. However, detailed studies have not been conducted on the uptake of peptide- or amino acid-chelated Fe into the plant or its transport between organs. Few studies have focused on the oligopeptide transporter (OPT) and drug/metabolite transporter (DMT) families. The possible roles of the recently discovered UMAMIT amino acid transporter family have not been studied in iron transport in plants yet. In this review, the transporter families responsible for the uptake and translocation of iron were summarized. Then, the roles of the OPT, DMT, and UMAMIT families in transporting iron-peptide and iron-amino acid complexes were discussed in detail.
Anahtar Kelime: Belge Türü: Makale Makale Türü: Derleme Erişim Türü: Erişime Açık
- Aksoy E, Yerlikaya BA, Ayten S, Abudureyimu B. 2018. Iron Uptake Mechanisms from the Rhizosphere in Plants. Turkish Journal of Agriculture-Food Science and Technology, 6(12): 1673-1683. DOI: https://doi.org/10.24925/turjaf.v6i12.1673- 1683.1326
- Aksoy E. 2014. Arabidopsis thaliana CARBOXYL-TERMINAL DOMAIN PHOSPHATASE-Like1 (CPL1) Mediates Responses to Iron Deficiency and Cadmium Toxicity. PhD Dissertation. Faculty of Agricultural and Life Sciences, Texas A&M University, College Station, TX-USA.
- Anjum NA, Umar S, Singh S, Nazar R, Khan NA. 2008. Sulfur assimilation and cadmium tolerance in plants. In: Anjum NA, Umar S, Singh S, Nazar R, Khan NA (editors). Sulfur assimilation and abiotic stress in plants. Springer. Netherlands. pp. 271-302. ISBN: 978-3-540-76326-0 (online). DOI: https://doi.org/10.1007/978-3-540-76326-0
- Aoyama T, Kobayashi T, Takahashi M, Nagasaka S, Usuda K, Kakei Y, Ishimaru Y, Nakanishi H, Mori S, Nishizawa NK. 2009. OsYSL18 is a rice iron (III)–deoxymugineic acid transporter specifically expressed in reproductive organs and phloem of lamina joints. Plant Molecular Biology, 70(6): 681-692. DOI: https://doi.org/10.1007/s11103-009-9500-3
- Arrivault S, Senger T, Krämer U. 2006. The Arabidopsis metal tolerance protein AtMTP3 maintains metal homeostasis by mediating Zn exclusion from the shoot under Fe deficiency and Zn oversupply. Plant Journal, 46(5): 861-879. DOI: https://doi.org/10.1111/j.1365-313X.2006.02746.x
- Banakar R, Alvarez Fernandez A, Díaz-Benito P, Abadia J, Capell T, Christou P. 2017. Phytosiderophores determine thresholds for iron and zinc accumulation in biofortified rice endosperm while inhibiting the accumulation of cadmium. Journal of Experimental Botany, 68(17): 4983-4995. DOI: https://doi.org/10.1093/jxb/erx304
- Bashir K, Inoue H, Nagasaka S, Takahashi M, Nakanishi H, Mori S, Nishizawa NK. 2006. Cloning and characterization of deoxymugineic acid synthase genes from graminaceous plants. Journal of Biological Chemistry, 281: 32395-32402. DOI: https://doi.org/10.1074/jbc.M604133200
- Bashir K, Ishimaru Y, Itai RN, Senoura T, Takahashi M, An G, Oikawa T, Ueda M, Sato A, Uozumi N, Nakanishi H. 2015. Iron deficiency regulated OsOPT7 is essential for iron homeostasis in rice. Plant Molecular Biology, 88(1-2): 165- 176. DOI: https://doi.org/10.1007/s11103-015-0315-0
- Bashir K, Ishimaru Y, Shimo H, Kakei Y, Senoura T, Takahashi R, Sato Y, Sato Y, Uozumi N, Nakanishi H, Nishizawa NK. 2011. Rice phenolics efflux transporter 2 (PEZ2) plays an important role in solubilizing apoplasmic iron. Soil Science and Plant Nutrition, 57(6): 803-812. DOI: https://doi.org/10.1080/00380768.2011.637305
- Besnard J, Pratelli R, Zhao C, Sonawala U, Collakova E, Pilot G, Okumoto S. 2016. UMAMIT14 is an amino acid exporter involved in phloem unloading in Arabidopsis roots. Journal of Experimental Botany, 67(22): 6385-6397. DOI: https://doi.org/10.1093/jxb/erw412
- Besnard J, Zhao C, Avice JC, Vitha S, Hyodo A, Pilot G, Okumoto S. 2018. Arabidopsis UMAMIT24 and 25 are amino acid exporters involved in seed loading. Journal of Experimental Botany, 69(21): 5221-5232. DOI: https://doi.org/10.1093/jxb/ery302
- Briat J F, Dubos C, Gaymard F. 2015. Iron nutrition, biomass production, and plant product quality. Trends in Plant Science, 20: 33-40. DOI: https://doi.org/10.1016/j.tplants.2014.07.005
- Cao J, Huang J, Yang Y, Hu X. 2011. Analyses of the oligopeptide transporter gene family in poplar and grape. BMC Genomics, 12(1): 1-16. DOI: https://doi.org/10.1186/1471-2164-12-465
- Castaings L, Caquot A, Loubet S, Curie C. 2016. The high- affinity metal transporters NRAMP1 and IRT1 team up to take up iron under sufficient metal provision. Scientific Reports, 6(1): 1-11. DOI: https://doi.org/10.1038/srep37222
- Cheng L, Yuan HY, Ren R, Zhao SQ, Han YP, Zhou QY, Ke DX, Wang YX, Wang L. 2016. Genome-wide identification, classification, and expression analysis of amino acid transporter gene family in Glycine max. Frontiers in Plant Science, 7:515. DOI: https://doi.org/10.3389/fpls.2016.00515
- Chowdhury R, Nallusamy S, Shanmugam V, Loganathan A, Muthurajan R, Sivathapandian SK, Paramasivam J, Duraialagaraja, S. 2022. Genome-wide understanding of evolutionary and functional relationships of rice Yellow Stripe-Like (YSL) transporter family in comparison with other plant species. Biologia, 77(1): 39-53. DOI: https://doi.org/10.1007/s11756-021-00924-5
- Chu HH, Conte SS, Chan Rodriguez D, Vasques K, Punshon T, Salt DE, Walker EL. 2013. Arabidopsis thaliana Yellow Stripe1-Like4 and Yellow Stripe1-Like6 localize to internal cellular membranes and are involved in metal ion homeostasis. Frontiers in Plant Science, 4: 283. DOI: https://doi.org/10.3389/fpls.2013.00283
- Clemens S. 2019. Metal ligands in micronutrient acquisition and homeostasis. Plant, Cell and Environment, 42(10): 2902- 2912. DOI: https://doi.org/10.1111/pce.13627
- Connolly EL, Campbell NH, Grotz N, Prichard CL, Guerinot ML. 2003. Overexpression of the FRO2 ferric chelate reductase confers tolerance to growth on low iron and uncovers posttranscriptional control. Plant Physiology, 133: 1102- 1110. DOI: https://doi.org/10.1104/pp.103.025122
- Connolly EL, Fett JP, Guerinot ML. 2002. Expression of the IRT1 metal transporter is controlled by metals at the levels of transcript and protein accumulation. Plant Cell, 14: 1347- 1357. DOI: https://doi.org/10.1105/tpc.001263
- Conte SS, Walker EL. 2012. Genetic and biochemical approaches for studying the yellow stripe-like transporter family in plants. Current Topics in Membranes, 69: 295-322. DOI: https://doi.org/10.1016/B978-0-12-394390-3.00011-2
- Cui M, Gu M, Lu Y, Zhang Y, Chen C, Ling HQ, Wu H. 2020. Glutamate synthase 1 is involved in iron deficiency response and long distance transportation in Arabidopsis. Journal of Integrative Plant Biology, 62(12): 1925-1941. DOI: https://doi.org/10.1111/jipb.12985
- Curie C, Panaviene Z, Loulergue C, Dellaporta SL, Briat JF, Walker EL. 2001. Maize yellow stripe1 encodes a membrane protein directly involved in Fe (III) uptake. Nature, 409(6818): 346-349. DOI: https://doi.org/10.1038/35053080
- Dey S, Regon P, Kar S, Panda SK. 2020. Chelators of iron and their role in plant’s iron management. Physiology and Molecular Biology of Plants, 26(8): 1541-1549. DOI: https://doi.org/10.1007/s12298-020-00841-y
- Dinesh A, Rathod R, Sreedhar, M. 2020. In silico Identification and Analysis of Iron (Fe) Transporters in Various Plant Species. Current Journal of Applied Science and Technology, 39(38): 14-23. doi: 10.9734/CJAST/2020/v39i3831091.
- Dinkeloo K, Boyd S, Pilot G. 2018. Update on amino acid transporter functions and on possible amino acid sensing mechanisms in plants. Seminars in Cell and Developmental Biology, 74: 105-113). DOI: https://doi.org/10.1016/ j.semcdb.2017.07.010
- Divol F, Couch D, Conéjéro G, Roschzttardtz H, Mari S, Curie C. 2013. The Arabidopsis YELLOW STRIPE LIKE4 and 6 transporters control iron release from the chloroplast. Plant Cell, 25(3): 1040-1055. DOI: https://doi.org/10.1105/ tpc.112.107672
- Durrett TP, Gassmann W, Rogers EE. 2007. The FRD3-mediated efflux of citrate into the root vasculature is necessary for efficient iron translocation. Plant Physiology, 144(1): 197- 205. DOI: https://doi.org/10.1104/pp.107.097162
- Eide D, Broderius M, Fett J, Guerinot ML. 1996. A novel iron- regulated metal transporter from plants identified by functional expression in yeast. Proceedings of the National Academy of Sciences, 93(11): 5624-5628. DOI: https://doi.org/10.1073/pnas.93.11.5624
- Eroglu S, Meier B, von Wirén N, Peiter E. 2016. The vacuolar manganese transporter MTP8 determines tolerance to iron deficiency-induced chlorosis in Arabidopsis. Plant Physiology, 170(2): 1030-1045. DOI: https://doi.org/10.1104/ pp.15.01194
- Gao F, Robe K, Bettembourg M, Navarro N, Rofidal V, Santoni V, Gaymard F, Vignols F, Roschzttardtz H, Izquierdo E, Dubos C. 2020. The transcription factor bHLH121 interacts with bHLH105 (ILR3) and its closest homologs to regulate iron homeostasis in Arabidopsis. Plant Cell, 32(2): 508-524. DOI: https://doi.org/10.1105/tpc.19.00541
- Green LS, Rogers EE. 2004. FRD3 controls iron localization in Arabidopsis. Plant Physiology, 136(1): 2523-2531. DOI: https://doi.org/10.1104/pp.104.045633
- Grillet L, Lan P, Li W, Mokkapati G, Schmidt W. 2018. IRON MAN is a ubiquitous family of peptides that control iron transport in plants. Nature Plants, 4(11): 953-963. DOI: https://doi.org/10.1038/s41477-018-0266-y
- Grillet L, Schmidt W. 2019. Iron acquisition strategies in land plants: Not so different after all. New Phytologist, 224(1): 11- 18. DOI: https://doi.org/10.1111/nph.16005
- Gross J, Stein RJ, Fett-Neto AG, Fett JP. 2003. Iron homeostasis related genes in rice. Genetics and Molecular Biology, 26(4): 477-497. DOI: https://doi.org/10.1590/S1415-47572003000400012
- Hanikenne M, Esteves SM, Fanara S, Rouached H. 2021. Coordinated homeostasis of essential mineral nutrients: a focus on iron. Journal of Experimental Botany, 72(6): 2136- 2153. DOI: https://doi.org/10.1093/jxb/eraa483
- Haydon MJ, Cobbett CS. 2007. A novel major facilitator superfamily protein at the tonoplast influences zinc tolerance and accumulation in Arabidopsis. Plant Physiology, 143(4): 1705-1719. DOI: https://doi.org/10.1104/pp.106.092015
- Haydon MJ, Kawachi M, Wirtz M, Hillmer S, Hell R, Krämer U. 2012. Vacuolar nicotianamine has critical and distinct roles under iron deficiency and for zinc sequestration in Arabidopsis. Plant Cell, 24(2): 724-737. DOI: https://doi.org/10.1105/tpc.111.095042
- Hell R, Stephan UW. 2003. Iron uptake, trafficking and homeostasis in plants. Planta, 216(4): 541-551. DOI: https://doi.org/10.1007/s00425-002-0920-4
- Hirayama T, Lei GJ, Yamaji N, Nakagawa N, Ma JF. 2018. The putative peptide gene FEP1 regulates iron deficiency response in Arabidopsis. Plant and Cell Physiology, 59(9): 1739-1752. DOI: https://doi.org/10.1093/pcp/pcy145
- Holt EM, Holt SL, Tucker WF, Asplund RO, Watson KJ. 1974. Preparation and properties of iron (III)-amino acid complexes. Iron (III)-alanine, a possible ferritin analog. Journal of the American Chemical Society, 96(8): 2621-2623. doi: 10.1021/ja00815a055
- Inoue H, Kobayashi T, Nozoye T, Takahashi M, Kakei Y, Suzuki K, Nakazono M, Nakanishi H, Mori S, Nishizawa NK. 2009. Rice OsYSL15 is an iron-regulated iron (III)-deoxymugineic acid transporter expressed in the roots and is essential for iron uptake in early growth of the seedlings. Journal of Biological Chemistry, 284(6): 3470-3479. DOI: https://doi.org/10.1074/ jbc.M806042200
- Ishimaru Y, Kakei Y, Shimo H, Bashir K, Sato Y, Sato Y, Uozumi N, Nakanishi H, Nishizawa NK. 2011. A rice phenolic efflux transporter is essential for solubilizing precipitated apoplasmic iron in the plant stele. Journal of Biological Chemistry, 286(28): 24649-24655. DOI: https://doi.org/10.1074/jbc.M111.221168
- Ishimaru Y, Masuda H, Bashir K, Inoue H, Tsukamoto T, Takahashi M, Nakanishi H, Aoki N, Hirose T, Ohsugi R, Nishizawa NK. 2010. Rice metal nicotianamine transporter, OsYSL2, is required for the long distance transport of iron and manganese. Plant Journal, 62(3): 379-390. DOI: https://doi.org/10.1111/j.1365-313X.2010.04158.x
- Jack DL, Yang NM, Saier MH. 2001. The drug/metabolite transporter superfamily. European Journal of Biochemistry, 268(13): 3620-3639. DOI: https://doi.org/10.1046/j.1432- 1327.2001.02265.x
- Jean ML, Schikora A, Mari S, Briat JF, Curie C. 2005. A loss of function mutation in AtYSL1 reveals its role in iron and nicotianamine seed loading. Plant Journal, 44(5): 769-782. DOI: https://doi.org/10.1111/j.1365-313X.2005.02569.x
- Jeong J, Connolly EL. 2009. Iron uptake mechanisms in plants: Functions of the FRO family of ferric reductases. Plant Science, 176: 709-714. DOI: https://doi.org/10.1016/ j.plantsci.2009.02.011
- Kakei Y, Ishimaru Y, Kobayashi T, Yamakawa T, Nakanishi H, Nishizawa NK. 2012. OsYSL16 plays a role in the allocation of iron. Plant Molecular Biology, 79(6): 583-594. DOI: https://doi.org/10.1007/s11103-012-9930-1
- Kakei Y, Yamaguchi I, Kobayashi T, Takahashi M, Nakanishi H, Yamakawa T, Nishizawa NK. 2009. A highly sensitive, quick and simple quantification method for nicotianamine and 2′- deoxymugineic acid from minimum samples using LC/ESI- TOF-MS achieves functional analysis of these components in plants. Plant and Cell Physiology, 50(11): 1988-1993. DOI: https://doi.org/10.1093/pcp/pcp141
- Kerkeb L, Krämer U. 2003. The Role of Free Histidine in Xylem Loading of Nickel in Alyssum lesbiacum and Brassica juncea. Plant Physiology, 131(2): 716-724. DOI: https://doi.org/10.1104/ pp102.010686 Kim JY, Loo EPI, Pang TY, Lercher M, Frommer WB, Wudick MM. 2021. Cellular export of sugars and amino acids: role in feeding other cells and organisms. Plant Physiology, 187(4): 1893-1914. DOI: https://doi.org/10.1093/plphys/kiab228
- Klatte M, Schuler M, Wirtz M, Fink-Straube C, Hell R, Bauer P. 2009. The Analysis of Arabidopsis Nicotianamine Synthase mutants reveals functions for nicotianamine in seed iron loading and iron deficiency responses. Plant Physiology, 150: 257-271. DOI: https://doi.org/10.1104/pp.109.136374
- Kobayashi T, Nishizawa NK. 2012. Iron uptake, translocation, and regulation in higher plants. Annual Review of Plant Biology. 63: 131-152. DOI: 10.1146/annurev-arplant- 042811-105522
- Kobayashi T, Nozoye T, Nishizawa NK. 2019. Iron transport and its regulation in plants. Free Radical Biology and Medicine, 133: 11- 20. DOI: https://doi.org/10.1016/j.freeradbiomed.2018.10.439
- Kobayashi T, Suzuki M, Inoue H, Itai RN, Takahashi M, Nakanishi H, Mori S, Nishizawa NK. 2005. Expression of iron-acquisition-related genes in iron-deficient rice is co- ordinately induced by partially conserved iron-deficiency- responsive elements. Journal of Experimental Botany. 56: 1305-1316. DOI: https://doi.org/10.1093/jxb/eri131
- Koike S, Inoue H, Mizuno D, Takahashi M, Nakanishi H, Mori S, Nishizawa NK. 2004. OsYSL2 is a rice metal nicotianamine transporter that is regulated by iron and expressed in the phloem. Plant Journal, 39(3): 415-424. DOI: https://doi.org/10.1111/j.1365-313X.2004.02146.x
- Kumar A, Kaur G, Goel P, Bhati KK, Kaur M, Shukla V, Pandey AK. 2019. Genome-wide analysis of oligopeptide transporters and detailed characterization of yellow stripe transporter genes in hexaploid wheat. Functional and Integrative Genomics, 19(1): 75-90. DOI: https://doi.org/10.1007/s10142-018-0629-5
- Ladwig F, Stahl M, Ludewig U, Hirner AA, Hammes UZ, Stadler R, Harter K, Koch W. 2012. Siliques are Red1 from Arabidopsis acts as a bidirectional amino acid transporter that is crucial for the amino acid homeostasis of siliques. Plant Physiology, 158(4): 1643-1655. DOI: https://doi.org/10.1104/ pp.111.192583
- Liu T, Zeng J, Xia K, Fan T, Li Y, Wang Y, Xu X, Zhang M. 2012. Evolutionary expansion and functional diversification of oligopeptide transporter gene family in rice. Rice, 5(1): 1- 14. DOI: https://doi.org/10.1186/1939-8433-5-12
- Liu X, Bush DR. 2006. Expression and transcriptional regulation of amino acid transporters in plants. Amino Acids, 30(2): 113-120. DOI: https://doi.org/10.1007/s00726-005-0248-z
- Lubkowitz M. 2011. The oligopeptide transporters: a small gene family with a diverse group of substrates and functions?. Molecular Plant, 4(3): 407-415. DOI: https://doi.org/10.1093/mp/ssr004
- Ma H, Cao X, Shi S, Li S, Gao J, Ma Y, Zhao Q, Chen Q. 2016. Genome-wide survey and expression analysis of the amino acid transporter superfamily in potato (Solanum tuberosum L.). Plant Physiology and Biochemistry, 107: 164-177. DOI: https://doi.org/10.1016/j.plaphy.2016.06.007
- Marschner H, Marschner P. 2011. Marschner’s mineral nutrition of higher plants. New York: Academic Press. ISBN: 978-0- 12-384905-2.
- Mendoza-Cózatl DG, Xie Q, Akmakjian GZ, Jobe TO, Patel A, Stacey MG, Song L, Demoin DW, Jurisson SS, Stacey G, Schroeder JI. 2014. OPT3 is a component of the iron- signaling network between leaves and roots and misregulation of OPT3 leads to an over-accumulation of cadmium in seeds. Molecular Plant, 7(9): 1455-1469. DOI: https://doi.org/10.1093/mp/ssu067
- Morel M, Crouzet J, Gravot A, Auroy P, Leonhardt N, Vavasseur A, Richaud P. 2009. AtHMA3, a P1B-ATPase allowing Cd/Zn/co/Pb vacuolar storage in Arabidopsis. Plant Physiology, 149(2): 894-904. DOI: https://doi.org/10.1104/ pp.108.130294
- Mori S, Nishizawa N, Hayashi H, Chino M, Yoshimura E, Ishihara J. 1991. Why are young rice plants highly susceptible to iron deficiency?. In: Chen Y, Hadar Y (editors). Iron Nutrition and Interactions in Plants. Developments in Plant and Soil Sciences. Dordrecht: Springer. pp. 175-188. ISBN: 978-94-011-3294-7 (online). DOI: 10.1007/978-94-011-3294-7_23
- Morrissey J, Baxter IR, Lee J, Li L, Lahner B, Grotz N, Kaplan J, Salt DE, Guerinot ML. 2009. The ferroportin metal efflux proteins function in iron and cobalt homeostasis in Arabidopsis. The Plant Cell, 21(10): 3326-3338. DOI: https://doi.org/10.1105/tpc.109.069401
- Müller B, Fastner A, Karmann J, Mansch V, Hoffmann T, Schwab W, Suter-Grotemeyer M, Rentsch D, Truernit E, Ladwig F, Bleckmann A. 2015. Amino acid export in developing Arabidopsis seeds depends on UmamiT facilitators. Current Biology, 25(23): 3126-3131. DOI: https://doi.org/10.1016/j.cub.2015.10.038
- Murgia I, Marzorati F, Vigani G, Morandini P. 2022. Plant iron nutrition: the long road from soil to seeds. Journal of Experimental Botany, 73(6): 1809-1824. DOI: https://doi.org/10.1093/jxb/erab531
- Nagasaka S, Takahashi M, Nakanishi-Itai R, Bashir K, Nakanishi H, Mori S, Nishizawa NK. 2009. Time course analysis of gene expression over 24 hours in Fe-deficient barley roots. Plant Molecular Biology, 69: 621-631. DOI: https://doi.org/10.1007/s11103-008-9443-0
- Nimmy MS, Kumar V, Suthanthiram B, Subbaraya U, Nagar R, Bharadwaj C, Jain PK, Krishnamurthy P. 2022. A systematic phylogenomic classification of the Multidrug and Toxic Compound Extrusion Transporter gene family in plants. Frontiers in Plant Science, 13: 774885. DOI: 10.3389/fpls.2022.774885
- Nishiyama R, Kato M, Nagata S, Yanagisawa S, Yoneyama T. 2012. Identification of Zn–nicotianamine and Fe–2′- deoxymugineic acid in the phloem sap from rice plants (Oryza sativa L.). Plant and Cell Physiology, 53(2): 381-390. DOI: https://doi.org/10.1093/pcp/pcr188
- Nozoye T, Nagasaka S, Kobayashi T, Sato Y, Uozumi N, Nakanishi H, Nishizawa NK. 2015. The phytosiderophore efflux transporter TOM2 is involved in metal transport in rice. Journal of Biological Chemistry, 290(46): 27688-27699. DOI: https://doi.org/10.1074/jbc.M114.635193
- Nozoye T, Nagasaka S, Kobayashi T, Takahashi M, Sato Y, Sato Y, Uozumi N, Nakanishi H, Nishizawa NK. 2011. Phytosiderophore efflux transporters are crucial for iron acquisition in graminaceous plants. Journal of Biological Chemistry, 286(7): 5446-5454. DOI: https://doi.org/10.1074/ jbc.M110.180026
- Okumoto S, Pilot G. 2011. Amino acid export in plants: a missing link in nitrogen cycling. Molecular Plant, 4(3): 453-463. DOI: https://doi.org/10.1093/mp/ssr003
- Ortiz-Lopez A, Chang HC, Bush DR. 2000. Amino acid transporters in plants. Biochimica et Biophysica Acta (BBA)- Biomembranes, 1465(1-2): 275-280. DOI: https://doi.org/ 10.1016/S0005-2736(00)00144-9
- Palmer CM, Guerinot ML. 2009. Facing the challenges of Cu, Fe and Zn homeostasis in plants. Nature Chemical Biology, 5(5): 333. DOI: https://doi.org/10.1038/nchembio.166
- Pike S, Patel A, Stacey G, Gassmann W. 2009. Arabidopsis OPT6 is an oligopeptide transporter with exceptionally broad substrate specificity. Plant and Cell Physiology, 50(11): 1923-1932. DOI: https://doi.org/10.1093/pcp/pcp136
- Pu Y, Yang D, Yin X, Wang Q, Chen Q, Yang Y, Yang Y. 2018. Genome-wide analysis indicates diverse physiological roles of the turnip (Brassica rapa var. rapa) oligopeptide transporters gene family. Plant Diversity, 40(2): 57-67. DOI: https://doi.org/10.1016/j.pld.2018.03.001
- Rai S, Singh PK, Mankotia S, Swain J, Satbhai SB. 2021. Iron homeostasis in plants and its crosstalk with copper, zinc, and manganese. Plant Stress, 1: 100008. DOI: https://doi.org/ 10.1016/j.stress.2021.100008
- Ranocha P, Dima O, Nagy R, Felten J, Corratgé-Faillie C, Novák O, Morreel K, Lacombe B, Martinez Y, Pfrunder S, Jin X. 2013. Arabidopsis WAT1 is a vacuolar auxin transport facilitator required for auxin homoeostasis. Nature Communications, 4(1): 1-9. DOI: https://doi.org/10.1038/ncomms3625
- Reddy IM, Mahoney AW. 1995. Solution visible difference spectral properties of Fe3+-L-amino acid complexes at pH 6.60. Journal of Agricultural and Food Chemistry, 43(6): 1436-1443. doi: 10.1021/jf00054a005
- Robinson NJ, Procter CM, Connolly EL, Guerinot ML. 1999. A ferric-chelate reductase for iron uptake from soils. Nature, 397(6721): 694. DOI: https://doi.org/10.1038/17800
- Rogers EE, Guerinot ML. 2002. FRD3, a member of the multidrug and toxin efflux family, controls iron deficiency responses in Arabidopsis. Plant Cell, 14(8): 1787-1799. DOI: https://doi.org/10.1105/tpc.001495
- Roschzttardtz H, Séguéla-Arnaud M, Briat JF, Vert G, Curie C. 2011. The FRD3 citrate effluxer promotes iron nutrition between symplastically disconnected tissues throughout Arabidopsis development. Plant Cell, 23(7): 2725-2737. DOI: https://doi.org/10.1105/tpc.111.088088
- Santi S, Schmidt W. 2009. Dissecting iron deficiency induced proton extrusion in Arabidopsis roots. New Phytologist, 183(4): 1072-1084. DOI: https://doi.org/10.1111/j.1469- 8137.2009.02908.x
- Schaaf G, Honsbein A, Meda AR, Kirchner S, Wipf D, von Wirén N, 2006. AtIREG2 encodes a tonoplast transport protein involved in iron-dependent nickel detoxification in Arabidopsis thaliana roots. Journal of Biological Chemistry, 281(35): 25532-25540. DOI: https://doi.org/10.1074/ jbc.M601062200
- Schaaf G, Ludewig U, Erenoglu BE, Mori S, Kitahara T, von Wirén N, 2004. ZmYS1 functions as a proton-coupled symporter for phytosiderophore-and nicotianamine-chelated metals. Journal of Biological Chemistry, 279(10): 9091-9096. DOI: https://doi.org/10.1074/jbc.M311799200
- Shojima S, Nishizawa NK, Fushiya S, Nozoe S, Kumashiro T, Nagata T, Ohata T, Mori S. 1989. Biosynthesis of nicotianamine in the suspension-cultured cells of tobacco (Nicotiana megalosiphon). Biology of Metals, 2(3): 142-145. DOI: https://doi.org/10.1007/BF01142552
- Stacey MG, Koh S, Becker J, Stacey G. 2002. AtOPT3, a member of the oligopeptide transporter family, is essential for embryo development in Arabidopsis. Plant Cell, 14(11): 2799-2811. DOI: https://doi.org/10.1105/tpc.005629
- Stacey MG, Osawa H, Patel A, Gassmann W, Stacey G. 2006. Expression analyses of Arabidopsis oligopeptide transporters during seed germination, vegetative growth and reproduction. Planta, 223(2): 291-305. DOI: https://doi.org/10.1007/ s00425-005-0087-x
- Stacey MG, Patel A, McClain WE, Mathieu M, Remley M, Rogers EE, Gassmann W, Blevins DG, Stacey G. 2008. The Arabidopsis AtOPT3 protein functions in metal homeostasis and movement of iron to developing seeds. Plant Physiology, 146(2): 589-601. DOI: 10.1104/pp.107.108183
- Su H, Chu Y, Bai J, Gong L, Huang J, Xu W, Zhang J, Qiu X, Xu J, Huang Z. 2019. Genome-wide identification and comparative analysis for OPT family genes in Panax ginseng and eleven flowering plants. Molecules, 24(1): 15. DOI: https://doi.org/10.3390/molecules24010015
- Takahashi M, Terada Y, Nakai I, Nakanishi H, Yoshimura E, Mori S, Nishizawa NK. 2003. Role of nicotianamine in the intracellular delivery of metals and plant reproductive development. Plant Cell, 15(6): 1263-1280. DOI: https://doi.org/10.1105/tpc.15.010256
- Tegeder M. 2012. Transporters for amino acids in plant cells: some functions and many unknowns. Current Opinion in Plant Biology, 15(3): 315-321. DOI: https://doi.org/10.1016/ j.pbi.2012.02.001
- Thundathil, RV, Holt EM, Holt SL, Watson KJ. 1977. Preparation and properties of iron (III)-amino acid complexes. 2. The crystal and molecular structure of monoclinic tri-. mu. 3-oxo- triaquohexakis (glycine) triiron (III) perchlorate. Journal of the American Chemical Society, 99(6): 1818-1823. DOI: https://doi.org/10.1021/ja00448a024
- Tsuchiya, H, Doki S, Takemoto M, Ikuta T, Higuchi T, Fukui K, Usuda Y, Tabuchi E, Nagatoishi S, Tsumoto K, Nishizawa T. 2016. Structural basis for amino acid export by DMT superfamily transporter YddG. Nature, 534(7607): 417-420. DOI: https://doi.org/10.1038/nature17991
- Tucker WF, Asplund RO, Holt SL. 1975. Preparation and properties of Fe3+-amino acid complexes: Crystalline complexes with aliphatic amino acids. Archives of Biochemistry and Biophysics, 166(2): 433-438. DOI: https://doi.org/10.1016/0003-9861(75)90406-3
- Ueno D, Rombola AD, Iwashita T, Nomoto K, Ma JF. 2007. Identification of two new phytosiderophores secreted by perennial grasses. New Phytologist, 174: 304–310. DOI: https://doi.org/10.1111/j.1469-8137.2007.02056.x
- Vasconcelos, MW, Li GW, Lubkowitz MA, Grusak MA. 2008. Characterization of the PT clade of oligopeptide transporters in rice. Plant Genome, 1(2): 77-88. DOI: https://doi.org/10.3835/plantgenome2007.10.0540
- Vert G, Barberon M, Zelazny E, Seguela M, Briat JF, Curie C. 2009. Arabidopsis IRT2 cooperates with the high-affinity iron uptake system to maintain iron homeostasis in root epidermal cells. Planta. 229: 1171-1179. DOI: https://doi.org/10.1007/s00425-009-0904-8
- Vert G, Grotz N, Dédaldéchamp F, Gaymard F, Guerinot ML, Briat JF, Curie C. 2002. IRT1, an Arabidopsis transporter essential for iron uptake from the soil and for plant growth. Plant Cell, 14(6): 1223-1233. DOI: https://doi.org/10.1105/ tpc.001388
- Wan Y, King R, Mitchell RA, Hassani-Pak K, Hawkesford MJ. 2017. Spatiotemporal expression patterns of wheat amino acid transporters reveal their putative roles in nitrogen transport and responses to abiotic stress. Scientific Reports, 7(1): 1-13. DOI: https://doi.org/10.1038/s41598-017-04473-3
- Wang C, Wang X, Li J, Guan J, Tan Z, Zhang Z, Shi G. 2022. Genome-wide identification and transcript analysis reveal potential roles of oligopeptide transporter genes in iron deficiency induced cadmium accumulation in peanut. Frontiers in Plant Science, 13: 894848. DOI: 10.3389/fpls.2022.894848
- Wang L, Bei X, Gao J, Li Y, Yan Y, Hu Y. 2016. The similar and different evolutionary trends of MATE family occurred between rice and Arabidopsis thaliana. BMC Plant Biology, 16(1): 1-19. DOI: https://doi.org/10.1186/s12870-016-0895-0
- Waters BM, Chu HH, DiDonato RJ, Roberts LA, Eisley RB, Lahner B, Salt DE, Walker EL. 2006. Mutations in Arabidopsis yellow stripe-like1 and yellow stripe-like3 reveal their roles in metal ion homeostasis and loading of metal ions in seeds. Plant Physiology, 141(4): 1446-1458. DOI: https://doi.org/10.1104/pp.106.082586
- White PJ, Brown PH. 2010. Plant nutrition for sustainable development and global health. Annals of Botany, 105(7): 1073-1080. DOI: https://doi.org/10.1093/aob/mcq085
- Wintz H, Fox T, Wu YY, Feng V, Chen W, Chang HS, Zhu T, Vulpe C. 2003. Expression profiles of Arabidopsis thaliana in mineral deficiencies reveal novel transporters involved in metal homeostasis. Journal of Biological Chemistry, 278(48): 47644-47653. DOI: https://doi.org/10.1074/jbc.M309338200
- Wipf D, Loqué D, Lalonde S, Frommer WB. 2012. Amino acid transporter inventory of the Selaginella genome. Frontiers in Plant Science, 3: 36. DOI: https://doi.org/ 10.3389/fpls.2012.00036
- Wu D, He G, Tian W, Saleem M, Li D, Huang Y, Meng L, He Y, Liu Y, He T. 2021. OPT gene family analysis of potato (Solanum tuberosum) responding to heavy metal stress: Comparative omics and co-expression networks revealed the underlying core templates and specific response patterns. International Journal of Biological Macromolecules, 188: 892-903. DOI: https://doi.org/10.1016/ j.ijbiomac.2021.07.183
- Wu M, Wu S, Chen Z, Dong Q, Yan H, Xiang Y. 2015. Genome- wide survey and expression analysis of the amino acid transporter gene family in poplar. Tree Genetics and Genomes, 11(4): 1-20. DOI: https://doi.org/10.1007/s11295- 015-0908-4
- Xiang Q, Shen K, Yu X, Zhao K, Gu Y, Zhang X, Chen X, Chen Q. 2017. Analysis of the oligopeptide transporter gene family in Ganoderma lucidum: structure, phylogeny, and expression patterns. Genome, 60(4): 293-302. DOI: https://doi.org/ 10.1139/gen-2016-012
- Xie Y, Zhao JL, Wang CW, Yu AX, Liu N, Chen L, Lin F, Xu HH. 2016. Glycinergic–fipronil uptake is mediated by an amino acid carrier system and induces the expression of amino acid transporter genes in Ricinus communis seedlings. Journal of Agricultural and Food Chemistry, 64(19): 3810- 3818. DOI: https://doi.org/10.1021/acs.jafc.5b06042
- Yang G, Wei Q, Huang H, Xia J. 2020. Amino Acid Transporters in Plant Cells: A Brief Review. Plants, 9(8): 967. DOI: https://doi.org/10.3390/plants9080967
- Yao X, Nie J, Bai R, Sui X. 2020. Amino Acid Transporters in Plants: Identification and Function. Plants, 9(8): 972. DOI: https://doi.org/10.3390/plants9080972
- Yokosho K, Yamaji N, Ueno D, Mitani N, Ma JF. 2009. OsFRDL1 is a citrate transporter required for efficient translocation of iron in rice. Plant Physiology, 149(1): 297- 305. DOI: https://doi.org/10.1104/pp.108.128132
- Yordem BK, Conte SS, Ma JF, Yokosho K, Vasques KA, Gopalsamy SN, Walker EL. 2011. Brachypodium distachyon as a new model system for understanding iron homeostasis in grasses: phylogenetic and expression analysis of Yellow Stripe-Like (YSL) transporters. Annals of Botany, 108(5): 821-833. DOI: https://doi.org/10.1093/aob/mcr200
- Zhai Z, Gayomba SR, Jung HI, Vimalakumari NK, Piñeros M, Craft E, Rutzke MA, Danku J, Lahner B, Punshon T, Guerinot ML. 2014. OPT3 is a phloem-specific iron transporter that is essential for systemic iron signaling and redistribution of iron and cadmium in Arabidopsis. Plant Cell, 26(5): 2249-2264. DOI: https://doi.org/10.1105/tpc.114.123737
- Zhang C, Lu W, Yang Y, Shen Z, Ma JF, Zheng L. 2018. OsYSL16 is required for preferential Cu distribution to floral organs in rice. Plant and Cell Physiology, 59(10): 2039-2051. DOI: https://doi.org/10.1093/pcp/pcy124
- Zhang YY, Stockmann R, Ng K, Ajlouni S. 2021. Opportunities for plant derived enhancers for iron, zinc, and calcium bioavailability: A review. Comprehensive Reviews in Food Science and Food Safety, 20(1): 652-685. DOI: https://doi.org/10.1111/1541-4337.12669
- Zhao C, Pratelli R, Yu S, Shelley B, Collakova E, Pilot G. 2021. Detailed characterization of the UMAMITs proteins provides insight into their evolution, amino acid transport properties, and role in the plant. Journal of Experimental Botany, 72(18): 6400-6417. doi: 10.1093/jxb/erab288
- Zhao H, Ma H, Yu L, Wang X, Zhao J. 2012. Genome-wide survey and expression analysis of amino acid transporter gene family in rice (Oryza sativa L.). PloS one, 7(11): e49210. DOI: https://doi.org/10.1371/journal.pone.0049210
APA | Aksoy E, MAQBOOL A, Yerlikaya B, Wahid F (2022). Bitki Peptit ve Amino Asit Taşıyıcılarının Demir Taşınmasındaki Görevleri. , 2646 - 2655. 10.24925/turjaf.v10isp1.2646-2655.5600 |
Chicago | Aksoy Emre,MAQBOOL AMIR,Yerlikaya Bayram Ali,Wahid Fazli Bitki Peptit ve Amino Asit Taşıyıcılarının Demir Taşınmasındaki Görevleri. (2022): 2646 - 2655. 10.24925/turjaf.v10isp1.2646-2655.5600 |
MLA | Aksoy Emre,MAQBOOL AMIR,Yerlikaya Bayram Ali,Wahid Fazli Bitki Peptit ve Amino Asit Taşıyıcılarının Demir Taşınmasındaki Görevleri. , 2022, ss.2646 - 2655. 10.24925/turjaf.v10isp1.2646-2655.5600 |
AMA | Aksoy E,MAQBOOL A,Yerlikaya B,Wahid F Bitki Peptit ve Amino Asit Taşıyıcılarının Demir Taşınmasındaki Görevleri. . 2022; 2646 - 2655. 10.24925/turjaf.v10isp1.2646-2655.5600 |
Vancouver | Aksoy E,MAQBOOL A,Yerlikaya B,Wahid F Bitki Peptit ve Amino Asit Taşıyıcılarının Demir Taşınmasındaki Görevleri. . 2022; 2646 - 2655. 10.24925/turjaf.v10isp1.2646-2655.5600 |
IEEE | Aksoy E,MAQBOOL A,Yerlikaya B,Wahid F "Bitki Peptit ve Amino Asit Taşıyıcılarının Demir Taşınmasındaki Görevleri." , ss.2646 - 2655, 2022. 10.24925/turjaf.v10isp1.2646-2655.5600 |
ISNAD | Aksoy, Emre vd. "Bitki Peptit ve Amino Asit Taşıyıcılarının Demir Taşınmasındaki Görevleri". (2022), 2646-2655. https://doi.org/10.24925/turjaf.v10isp1.2646-2655.5600 |
APA | Aksoy E, MAQBOOL A, Yerlikaya B, Wahid F (2022). Bitki Peptit ve Amino Asit Taşıyıcılarının Demir Taşınmasındaki Görevleri. Türk Tarım - Gıda Bilim ve Teknoloji dergisi, 10(sp1), 2646 - 2655. 10.24925/turjaf.v10isp1.2646-2655.5600 |
Chicago | Aksoy Emre,MAQBOOL AMIR,Yerlikaya Bayram Ali,Wahid Fazli Bitki Peptit ve Amino Asit Taşıyıcılarının Demir Taşınmasındaki Görevleri. Türk Tarım - Gıda Bilim ve Teknoloji dergisi 10, no.sp1 (2022): 2646 - 2655. 10.24925/turjaf.v10isp1.2646-2655.5600 |
MLA | Aksoy Emre,MAQBOOL AMIR,Yerlikaya Bayram Ali,Wahid Fazli Bitki Peptit ve Amino Asit Taşıyıcılarının Demir Taşınmasındaki Görevleri. Türk Tarım - Gıda Bilim ve Teknoloji dergisi, vol.10, no.sp1, 2022, ss.2646 - 2655. 10.24925/turjaf.v10isp1.2646-2655.5600 |
AMA | Aksoy E,MAQBOOL A,Yerlikaya B,Wahid F Bitki Peptit ve Amino Asit Taşıyıcılarının Demir Taşınmasındaki Görevleri. Türk Tarım - Gıda Bilim ve Teknoloji dergisi. 2022; 10(sp1): 2646 - 2655. 10.24925/turjaf.v10isp1.2646-2655.5600 |
Vancouver | Aksoy E,MAQBOOL A,Yerlikaya B,Wahid F Bitki Peptit ve Amino Asit Taşıyıcılarının Demir Taşınmasındaki Görevleri. Türk Tarım - Gıda Bilim ve Teknoloji dergisi. 2022; 10(sp1): 2646 - 2655. 10.24925/turjaf.v10isp1.2646-2655.5600 |
IEEE | Aksoy E,MAQBOOL A,Yerlikaya B,Wahid F "Bitki Peptit ve Amino Asit Taşıyıcılarının Demir Taşınmasındaki Görevleri." Türk Tarım - Gıda Bilim ve Teknoloji dergisi, 10, ss.2646 - 2655, 2022. 10.24925/turjaf.v10isp1.2646-2655.5600 |
ISNAD | Aksoy, Emre vd. "Bitki Peptit ve Amino Asit Taşıyıcılarının Demir Taşınmasındaki Görevleri". Türk Tarım - Gıda Bilim ve Teknoloji dergisi 10/sp1 (2022), 2646-2655. https://doi.org/10.24925/turjaf.v10isp1.2646-2655.5600 |