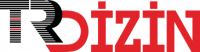
Osteoporoz Epigenetiği
Yıl: 2023 Cilt: 29 Sayı: 1 Sayfa Aralığı: 1 - 9 Metin Dili: Türkçe DOI: 10.4274/tod.galenos.2022.80148 İndeks Tarihi: 03-06-2023
Osteoporoz Epigenetiği
Öz: Osteoporoz (OP), düşük kemik kütlesi ve kemik dokusunun mikromimarisinin bozulması, bunun sonucunda kemik kırılganlığında ve kırılma eğiliminde artış ile karakterize sistemik bir hastalıktır. Prenatal dönem dahil olmak üzere yaşamın erken dönemindeki çevresel faktörler, yaşamın sonraki dönemlerinde kemik kütlesini ve dolayısıyla OP riskini etkileyebilir. Epigenetik esas olarak transkripsiyon sonrası düzenleyici bir rol oynar ve biyolojik sinyal düzenleyici yolakta önemli işlevlere sahiptir. Epigenetik mekanizmalar, kemik oluşumu ve kemik erimesinden sorumlu olan kemik hücrelerinin, osteoblastların ve osteoklastların farklılaşmasında önemli rol oynar. Birkaç çalışma, OP’lu hastalarda bazı farklı şekilde metillenmiş genler göstermiştir. Anormal epigenetik düzenleme, OP gibi bir dizi kemik metabolizması ile ilgili hastalığa yol açabilir. Bunlar, osteoblast farklılaşmasının önemli bir düzenleyicisi olan Wnt yoluna ait genleri ve iskeletin gelişiminde rol oynayan diğer genleri içerir. Benzer şekilde, bu hastalarda bazı MikroRNA’lar farklı şekilde eksprese edilebilir. Ancak, bu sonuçların diğer kohortlarda tekrarlanması önerilmektedir. Genomdan farklı olarak, epigenom hücreye özgüdür ve yaşlanma ve çevresel faktörlerle değişir. Bu nedenle, epigenetik epidemiyoloji çalışmalarının tasarımı ve yorumlanması birtakım pratik zorluklar doğurmaktadır. Epigenetik mekanizmalar, kemik oluşumu ve kemik erimesinden sorumlu olan kemik hücrelerinin, osteoblastların ve osteoklastların farklılaşmasında önemli rol oynamaktadır.
Anahtar Kelime: Epigenetics of Osteoporosis
Öz: Osteoporosis (OP) is a systemic disease characterized by low bone mass and deterioration of the microarchitecture of bone tissue, resulting in increased bone fragility and fracture tendency. Environmental factors early in life, including the prenatal period, can affect bone mass later in life and thus the risk of OP. Epigenetics mainly plays a post-transcriptional regulatory role and has important functions in the biological signal- regulatory pathway. Epigenetic mechanisms play an important role in the differentiation of bone cells, osteoblasts, and osteoclasts, which are responsible for bone formation and bone resorption. Several studies have shown some differentially methylated genes in patients with OP. The abnormal epigenetic regulation can lead to a number of bone metabolism-related diseases such as OP. These include genes for theWnt pathway, an important regulator of osteoblast differentiation, and other genes involved in skeletal development. Similarly, some MicroRNA’s may be differentially expressed in these patients. However, it is recommended that these results should be replicated in other cohorts. Unlike the genome, the epigenome is cell-specific and changes with aging and environmental factors. Therefore, the design and interpretation of epigenetic epidemiology studies pose a number of practical challenges. Epigenetic mechanisms play an important role in the differentiation of bone cells, osteoblasts, and osteoclasts, which are responsible for bone formation and bone resorption.
Anahtar Kelime: Belge Türü: Makale Makale Türü: Derleme Erişim Türü: Erişime Açık
- 1. Meunier P, Aaron J, Edouard C, Vignon G. Osteoporosis and the replacement of cell populations of the marrow by adipose tissue. A quantitative study of 84 iliac bone biopsies. Clin Orthop Relat Res 1971;80:147-54.
- 2. Justesen J, Stenderup K, Ebbesen EN, Mosekilde L, Steiniche T, Kassem M. Adipocyte tissue volume in bone marrow is increased with aging and in patients with osteoporosis. Biogerontology 2001;2:165-71.
- 3. Owen M. Marrow stromal stem cells. J Cell Sci Suppl 1988;10:63- 76.
- 4. Pittenger MF, Mackay AM, Beck SC, Jaiswal RK, Douglas R, Mosca JD, et al. Multilineage potential of adult human mesenchymal stem cells. Science 1999;284:143-7.
- 5. Moore LD, Le T, Fan G. DNA methylation and its basic function. Neuropsychopharmacology 2013;38:23-38.
- 6. Yang S, Duan X. Epigenetics, Bone Remodeling and Osteoporosis. Curr Stem Cell Res Ther 2016.
- 7. Letarouilly JG, Broux O, Clabaut A. New insights into the epigenetics of osteoporosis. Genomics 2019;111:793-8.
- 8. Xu F, Liu J, Na L, Chen L. Roles of Epigenetic Modifications in the Differentiation and Function of Pancreatic β-Cells. Front Cell Dev Biol 2020;8:748.
- 9. Niu YD, Lin YH, Zhang HQ, Tao T and Xu LT. Research progress on the role of DNA methylation in bone metabolism regulation and osteoporosis. Chin Bull Life Sci 2020;2:162-169.
- 10. Zhang J, Tu Q, Bonewald LF, He X, Stein G, Lian J, et al. Effects of miR-335-5p in modulating osteogenic differentiation by specifically downregulating Wnt antagonist DKK1. J Bone Miner Res 2011;26:1953-63.
- 11. Raje MM, Ashma R. Epigenetic regulation of BMP2 gene in osteoporosis: a DNA methylation study. Mol Biol Rep 2019;46:1667-74.
- 12. Cao Y, Wang B, Wang D, Zhan D, Mai C, Wang P, et al. Expression of Sclerostin in Osteoporotic Fracture Patients Is Associated with DNA Methylation in the CpG Island of the SOST Gene. Int J Genomics 2019;2019:7076513.
- 13. Licini C, Vitale-Brovarone C, Mattioli-Belmonte M. Collagen and non-collagenous proteins molecular crosstalk in the pathophysiology of osteoporosis. Cytokine Growth Factor Rev 2019;49:59-69.
- 14. Wu F, Jiao J, Liu F, Yang Y, Zhang S, Fang Z, et al. Hypermethylation of Frizzled1 is associated with Wnt/β-catenin signaling inactivation in mesenchymal stem cells of patients with steroid-associated osteonecrosis. Exp Mol Med 2019;51:1-9.
- 15. Behera J, George AK, Voor MJ, Tyagi SC, Tyagi N. Hydrogen sulfide epigenetically mitigates bone loss through OPG/RANKL regulation during hyperhomocysteinemia in mice. Bone 2018;114:90-108.
- 16. Wang P, Cao Y, Zhan D, Wang D, Wang B, Liu Yet al. Influence of DNA methylation on the expression of OPG/RANKL in primary osteoporosis. Int J Med Sci 2018;15:1480-5
- 17. Thaler R, Agsten M, Spitzer S, Paschalis EP, Karlic H, Klaushofer K, et al. Homocysteine suppresses the expression of the collagen cross-linker lysyl oxidase involving IL-6, Fli1, and epigenetic DNA methylation. J Biol Chem 2011;286:5578-88.
- 18. Penolazzi L, Lambertini E, Giordano S, Sollazzo V, Traina G, del Senno L, et al. Methylation analysis of the promoter F of estrogen receptor alpha gene: effects on the level of transcription on human osteoblastic cells. J Steroid Biochem Mol Biol 2004;91:1-9.
- 19. Li H, Xie H, Liu W, Hu R, Huang B, Tan YF, et al. A novel microRNA targeting HDAC5 regulates osteoblast differentiation in mice and contributes to primary osteoporosis in humans. J Clin Invest 2009;119:3666-77.
- 20. Jintaridth P, Tungtrongchitr R, Preutthipan S, Mutirangura A. Hypomethylation of Alu elements in post-menopausal women with osteoporosis. PLoS One 2013;8:e70386.
- 21. Ren J, Huang D, Li R, Wang W, Zhou C. Control of mesenchymal stem cell biology by histone modifications. Cell Biosci 2020;10:11.
- 22. Longbotham JE, Zhang MY, Fujimori DG. Domain cross-talk in regulation of histone modifications: Molecular mechanisms and targeting opportunities. Curr Opin Chem Biol 2020;57:105-13.
- 23. Rahman MM, Kukita A, Kukita T, Shobuike T, Nakamura T, Kohashi O. Two histone deacetylase inhibitors, trichostatin A and sodium butyrate, suppress differentiation into osteoclasts but not into macrophages. Blood 2003;101:3451-9.
- 24. Hesse E, Saito H, Kiviranta R, Correa D, Yamana K, Neff L, et al. Zfp521 controls bone mass by HDAC3-dependent attenuation of Runx2 activity. J Cell Biol 2010;191:1271-83.
- 25. Jeon EJ, Lee KY, Choi NS, Lee MH, Kim HN, Jin YH, et al. Bone morphogenetic protein-2 stimulates Runx2 acetylation. J Biol Chem 2006;281:16502-11.
- 26. Kim HN, Lee JH, Bae SC, Ryoo HM, Kim HH, Ha H, et al. Histone deacetylase inhibitor MS-275 stimulates bone formation in part by enhancing Dhx36-mediated TNAP transcription. J Bone Miner Res 2011;26:2161-73.
- 27. Pham L, Kaiser B, Romsa A, Schwarz T, Gopalakrishnan R, Jensen ED, et al. HDAC3 and HDAC7 have opposite effects on osteoclast differentiation. J Biol Chem 2011;286:12056-65.
- 28. Fu Y, Zhang P, Ge J, Cheng J, Dong W, Yuan H, et al. Histone deacetylase 8 suppresses osteogenic differentiation of bone marrow stromal cells by inhibiting histone H3K9 acetylation and RUNX2 activity. Int J Biochem Cell Biol 2014;54:68-77.
- 29. Edwards JR, Perrien DS, Fleming N, Nyman JS, Ono K, Connelly L, et al. Silent information regulator (Sir)T1 inhibits NF-κB signaling to maintain normal skeletal remodeling. J Bone Miner Res 2013;28:960-9.
- 30. Nagai K, Matsushita T, Matsuzaki T, Takayama K, Matsumoto T, Kuroda R, et al. Depletion of SIRT6 causes cellular senescence, DNA damage, and telomere dysfunction in human chondrocytes. Osteoarthritis Cartilage 2015;23:1412-20.
- 31. Sun X, Yuan Y, Xiao Y, Lu Q, Yang L, Chen C, Guo Q. Long non coding RNA, Bmcob, regulates osteoblastic differentiation of bone marrow mesenchymal stem cells. Biochem Biophys Res Commun 2018;506:536-42.
- 32. Fan Z, Yamaza T, Lee JS, Yu J, Wang S, Fan G, et al. BCOR regulates mesenchymal stem cell function by epigenetic mechanisms. Nat Cell Biol 2009;11:1002-9.
- 33. Qi Q, Wang Y, Wang X, Yang J, Xie Y, Zhou J, et al. Histone demethylase KDM4A regulates adipogenic and osteogenic differentiation via epigenetic regulation of C/EBPα and canonical Wnt signaling. Cell Mol Life Sci 2020;77:2407-21.
- 34. Ye L, Fan Z, Yu B, Chang J, Al Hezaimi K, Zhou X, et al. Histone demethylases KDM4B and KDM6B promotes osteogenic differentiation of human MSCs. Cell Stem Cell 2012;11:50-61.
- 35. Flowers S, Beck GR Jr, Moran E. Transcriptional activation by pRB and its coordination with SWI/SNF recruitment. Cancer Res 2010;70:8282-7.
- 36. Hoang M, Kim JJ, Kim Y, Tong E, Trammell B, Liu Y, et al. Alcohol induced suppression of KDM6B dysregulates the mineralization potential in dental pulp stem cells. Stem Cell Res 2016;17:111-21.
- 37. Yang X, Wang G, Wang Y, Zhou J, Yuan H, Li X, et al. Histone demethylase KDM7A reciprocally regulates adipogenic and osteogenic differentiation via regulation of C/EBPα and canonical Wnt signalling. J Cell Mol Med 2019;23:2149-62.
- 38. Chen LL, Yang L. Regulation of circRNA biogenesis. RNA Biol 2015;12:381-8.
- 39. Ge W, Shi L, Zhou Y, Liu Y, Ma GE, Jiang Y, et al. Inhibition of osteogenic differentiation of human adipose-derived stromal cells by retinoblastoma binding protein 2 repression of RUNX2- activated transcription. Stem Cells 2011;29:1112-25.
- 40. Liu H, Liu Q, Wu XP, He HB, Fu L. MiR-96 regulates bone metabolism by targeting osterix. Clin Exp Pharmacol Physiol 2018;45:602-13.
- 41. Yang S, Duan X. Epigenetics, Bone Remodeling and Osteoporosis. Curr Stem Cell Res Ther 2016.
- 42. Liang WC, Fu WM, Wang YB, Sun YX, Xu LL, Wong CW, et al. H19 activates Wnt signaling and promotes osteoblast differentiation by functioning as a competing endogenous RNA. Sci Rep 2016;6:20121.
- 43. Hoang M, Kim JJ, Kim Y, Tong E, Trammell B, Liu Y, et al. Alcohol induced suppression of KDM6B dysregulates the mineralization potential in dental pulp stem cells. Stem Cell Res 2016;17:111-21.
- 44. Xia W, Zhuang L, Deng X, Hou M. Long noncoding RNA p21 modulates cellular senescence via the Wnt/β catenin signaling pathway in mesenchymal stem cells. Mol Med Rep 2017;16:7039-47.
- 45. Zhu J, Wang Y, Yu W, Xia K, Huang Y, Wang J, et al. Long Noncoding RNA: Function and Mechanism on Differentiation of Mesenchymal Stem Cells and Embryonic Stem Cells. Curr Stem Cell Res Ther 2019;14:259-67.
- 46. Chen J, Jia YS, Liu GZ, Sun Q, Zhang F, Ma S, et al. Role of LncRNA TUG1 in intervertebral disc degeneration and nucleus pulposus cells via regulating Wnt/β-catenin signaling pathway. Biochem Biophys Res Commun 2017;491:668-74.
- 47. Zhang W, Dong R, Diao S, Du J, Fan Z, Wang F. Differential long noncoding RNA/mRNA expression profiling and functional network analysis during osteogenic differentiation of human bone marrow mesenchymal stem cells. Stem Cell Res Ther 2017;8:30.
- 48. Tong X, Gu PC, Xu SZ, Lin XJ. Long non-coding RNA-DANCR in human circulating monocytes: a potential biomarker associated with postmenopausal osteoporosis. Biosci Biotechnol Biochem 2015;79:732-7.
- 49. Wei B, Wei W, Zhao B, Guo X, Liu S. Long non-coding RNA HOTAIR inhibits miR-17-5p to regulate osteogenic differentiation and proliferation in non-traumatic osteonecrosis of femoral head. PLoS One 2017;12:e0169097.
- 50. Che W, Dong Y, Quan HB. RANKL inhibits cell proliferation by regulating MALAT1 expression in a human osteoblastic cell line hFOB 1.19. Cell Mol Biol (Noisy-le-grand) 2015;61:7-14.
- 51. Weng J, Peng W, Zhu S, Chen S. Long Noncoding RNA Sponges miR-454 to Promote Osteogenic Differentiation in Maxillary Sinus Membrane Stem Cells. Implant Dent 2017;26:178-86.
- 52. Xu Y, Wang S, Tang C, Chen W. Upregulation of long non coding RNA HIF 1α-anti-sense 1 induced by transforming growth factor-β-mediated targeting of sirtuin 1 promotes osteoblastic differentiation of human bone marrow stromal cells. Mol Med Rep 2015;12:7233-8.
- 53. Wang Q, Li Y, Zhang Y, Ma L, Lin L, Meng J, Jiang L, Wang L, Zhou P, Zhang Y. LncRNA MEG3 inhibited osteogenic differentiation of bone marrow mesenchymal stem cells from postmenopausal osteoporosis by targeting miR-133a-3p. Biomed Pharmacother 2017;89:1178-86.
- 54. Feng L, Shi L, Lu YF, Wang B, Tang T, Fu WM, et al. Linc-ROR Promotes Osteogenic Differentiation of Mesenchymal Stem Cells by Functioning as a Competing Endogenous RNA for miR-138 and miR-145. Mol Ther Nucleic Acids 2018;11:345-53.
- 55. Xiao T, Liu L, Li H, Sun Y, Luo H, Li T, et al. Long Noncoding RNA ADINR Regulates Adipogenesis by Transcriptionally Activating C/ EBPα. Stem Cell Reports 2015;5:856-65.
- 56. Zhu XX, Yan YW, Chen D, Ai CZ, Lu X, Xu SS, et al. Long non-coding RNA HoxA-AS3 interacts with EZH2 to regulate lineage commitment of mesenchymal stem cells. Oncotarget 2016;7:63561-70
- 57. Yang L, Li Y, Gong R, Gao M, Feng C, Liu T, et al. The Long Non-coding RNA-ORLNC1 Regulates Bone Mass by Directing Mesenchymal Stem Cell Fate. Mol Ther 2019;27:394-410.
- 58. Li CJ, Xiao Y, Yang M, Su T, Sun X, Guo Q, Huang Y, Luo XH. Long noncoding RNA Bmncr regulates mesenchymal stem cell fate during skeletal aging. J Clin Invest 2018;128:5251-66.
- 59. Wang Q, Cai J, Cai XH, Chen L. miR-346 regulates osteogenic differentiation of human bone marrow-derived mesenchymal stem cells by targeting the Wnt/β-catenin pathway. PLoS One 2013;8:e72266.
- 60. Seeliger C, Karpinski K, Haug AT, Vester H, Schmitt A, Bauer JS, et al. Five freely circulating miRNAs and bone tissue miRNAs are associated with osteoporotic fractures. J Bone Miner Res 2014;29:1718-28.
- 61. Garmilla-Ezquerra P, Sañudo C, Delgado-Calle J, Pérez-Nuñez MI, Sumillera M, Riancho JA. Analysis of the bone microRNome in osteoporotic fractures. Calcif Tissue Int 2015;96:30-7.62. Hernandez CJ, Beaupré GS, Carter DR. A theoretical analysis of the relative influences of peak BMD, age-related bone loss and menopause on the development of osteoporosis. Osteoporos Int 2003;14:843-7.
- 62. Hernandez CJ, Beaupré GS, Carter DR. A theoretical analysis of the relative influences of peak BMD, age-related bone loss and menopause on the development of osteoporosis. Osteoporos Int 2003;14:843-7.
- 63. Ohta H, Kuroda T, Onoe Y, Nakano C, Yoshikata R, Ishitani K, et al. Familial correlation of bone mineral density, birth data and lifestyle factors among adolescent daughters, mothers and grandmothers. J Bone Miner Metab 2010;28:690-5.
- 64. Cvijetić Avdagić S, Colić Barić I, Keser I, Rumbak I, Šatalić Z. Influence of heredity and environment on peak bone density: a review of studies in Croatia. Arh Hig Rada Toksikol 2012;63:11-6.
- 65. Medina-Gomez C, Kemp JP, Estrada K, Eriksson J, Liu J, Reppe S, et al. Meta-analysis of genome-wide scans for total body BMD in children and adults reveals allelic heterogeneity and age-specific effects at the WNT16 locus. PLoS Genet 2012;8:e1002718.
- 66. Harvey N, Dennison E, Cooper C. Osteoporosis: a lifecourse approach. J Bone Miner Res 2014;29:1917-25.
- 67. Harvey NC, Mahon PA, Robinson SM, Nisbet CE, Javaid MK, Crozier SR, et al. Different indices of fetal growth predict bone size and volumetric density at 4 years of age. J Bone Miner Res 2010;25:920-7.
- 68. Baird J, Kurshid MA, Kim M, Harvey N, Dennison E, Cooper C. Does birthweight predict bone mass in adulthood? A systematic review and meta-analysis. Osteoporos Int 2011;22:1323-34.
- 69. Zhu K, Whitehouse AJ, Hart PH, Kusel M, Mountain J, et al. Maternal vitamin D status during pregnancy and bone mass in offspring at 20 years of age: a prospective cohort study. J Bone Miner Res 2014;29:1088-95.
- 70. Holroyd C, Harvey N, Dennison E, Cooper C. Epigenetic influences in the developmental origins of osteoporosis. Osteoporos Int 2012;23:401-10.
- 71. Harvey NC, Lillycrop KA, Garratt E, Sheppard A, McLean C, Burdge G, et al. Evaluation of methylation status of the eNOS promoter at birth in relation to childhood bone mineral content. Calcif Tissue Int 2012;90:120-7.
- 72. Harvey NC, Sheppard A, Godfrey KM, McLean C, Garratt E, Ntani G, et al. Childhood bone mineral content is associated with methylation status of the RXRA promoter at birth. J Bone Miner Res 2014;29:600-7.
- 73. Delgado-Calle J, Fernández AF, Sainz J, Zarrabeitia MT, Sañudo C, García-Renedo R, et al. Genome-wide profiling of bone reveals differentially methylated regions in osteoporosis and osteoarthritis. Arthritis Rheum 2013;65:197-205.
- 74. García-Ibarbia C, Delgado-Calle J, Casafont I, Velasco J, Arozamena J, Pérez-Núñez MI, et al. Contribution of genetic and epigenetic mechanisms to Wnt pathway activity in prevalent skeletal disorders. Gene 2013;532:165-72.
- 75. Reppe S, Noer A, Grimholt RM, Halldórsson BV, Medina-Gomez C, Gautvik VT, et al. Methylation of bone SOST, its mRNA, and serum sclerostin levels correlate strongly with fracture risk in postmenopausal women. J Bone Miner Res 2015;30:249-56.
- 76. Delgado-Calle J, Garmilla P, Riancho JA. Do epigenetic marks govern bone mass and homeostasis? Curr Genomics 2012;13:252-63.
- 77. Lian JB, Stein GS, van Wijnen AJ, Stein JL, Hassan MQ, Gaur T, et al. MicroRNA control of bone formation and homeostasis. Nat Rev Endocrinol 2012;8:212-27.
- 78. Garmilla-Ezquerra P, Sañudo C, Delgado-Calle J, Pérez-Nuñez MI, Sumillera M, Riancho JA. Analysis of the bone microRNome in osteoporotic fractures. Calcif Tissue Int 2015;96:30-7.
- 79. Li H, Xie H, Liu W, Hu R, Huang B, Tan YF, et al. A novel microRNA targeting HDAC5 regulates osteoblast differentiation in mice and contributes to primary osteoporosis in humans. J Clin Invest 2009;119:3666-77.
- 80. Heijmans BT, Mill J. Commentary: The seven plagues of epigenetic epidemiology. Int J Epidemiol 2012;41:74-8.
- 81. Liang L, Cookson WO. Grasping nettles: cellular heterogeneity and other confounders in epigenome-wide association studies. Hum Mol Genet 2014;23.
- 82. Jaffe AE, Irizarry RA. Accounting for cellular heterogeneity is critical in epigenome-wide association studies. Genome Biol 2014;15:R31.
- 83. Smallwood SA, Lee HJ, Angermueller C, Krueger F, Saadeh H, Peat J, et al. Single-cell genome-wide bisulfite sequencing for assessing epigenetic heterogeneity. Nat Methods 2014;11:817-20.
- 84. Nagarajan A, Roden C, Wajapeyee N. Reduced representation bisulfite sequencing to identify global alteration of DNA methylation. Methods Mol Biol 2014;1176:23-31.
- 85. Sandoval J, Heyn H, Moran S, Serra-Musach J, Pujana MA, Bibikova M, et al. Validation of a DNA methylation microarray for 450,000 CpG sites in the human genome. Epigenetics 2011;6:692-702.
- 86. Riancho JA. Epigenetics of Osteoporosis: Critical Analysis of Epigenetic Epidemiology Studies. Curr Genomics 2015;16:405- 10.
- 87. Tural S, Kara N, Alaylı G. Osteoporoz genetiği. Türk Osteoporoz Dergisi 2011;17:100-9.
- 88. Xu F, Li W, Yang X, Na L, Chen L, Liu G. The Roles of Epigenetics Regulation in Bone Metabolism and Osteoporosis. Front Cell Dev Biol 2021;8:619301.
- 89. Letarouilly JG, Broux O, Clabaut A. New insights into the epigenetics of osteoporosis. Genomics 2019;111:793-8.
APA | tural s, TEKCAN E, Tural E (2023). Osteoporoz Epigenetiği. , 1 - 9. 10.4274/tod.galenos.2022.80148 |
Chicago | tural sengul,TEKCAN ESRA,Tural Ercan Osteoporoz Epigenetiği. (2023): 1 - 9. 10.4274/tod.galenos.2022.80148 |
MLA | tural sengul,TEKCAN ESRA,Tural Ercan Osteoporoz Epigenetiği. , 2023, ss.1 - 9. 10.4274/tod.galenos.2022.80148 |
AMA | tural s,TEKCAN E,Tural E Osteoporoz Epigenetiği. . 2023; 1 - 9. 10.4274/tod.galenos.2022.80148 |
Vancouver | tural s,TEKCAN E,Tural E Osteoporoz Epigenetiği. . 2023; 1 - 9. 10.4274/tod.galenos.2022.80148 |
IEEE | tural s,TEKCAN E,Tural E "Osteoporoz Epigenetiği." , ss.1 - 9, 2023. 10.4274/tod.galenos.2022.80148 |
ISNAD | tural, sengul vd. "Osteoporoz Epigenetiği". (2023), 1-9. https://doi.org/10.4274/tod.galenos.2022.80148 |
APA | tural s, TEKCAN E, Tural E (2023). Osteoporoz Epigenetiği. Türk Osteoporoz Dergisi, 29(1), 1 - 9. 10.4274/tod.galenos.2022.80148 |
Chicago | tural sengul,TEKCAN ESRA,Tural Ercan Osteoporoz Epigenetiği. Türk Osteoporoz Dergisi 29, no.1 (2023): 1 - 9. 10.4274/tod.galenos.2022.80148 |
MLA | tural sengul,TEKCAN ESRA,Tural Ercan Osteoporoz Epigenetiği. Türk Osteoporoz Dergisi, vol.29, no.1, 2023, ss.1 - 9. 10.4274/tod.galenos.2022.80148 |
AMA | tural s,TEKCAN E,Tural E Osteoporoz Epigenetiği. Türk Osteoporoz Dergisi. 2023; 29(1): 1 - 9. 10.4274/tod.galenos.2022.80148 |
Vancouver | tural s,TEKCAN E,Tural E Osteoporoz Epigenetiği. Türk Osteoporoz Dergisi. 2023; 29(1): 1 - 9. 10.4274/tod.galenos.2022.80148 |
IEEE | tural s,TEKCAN E,Tural E "Osteoporoz Epigenetiği." Türk Osteoporoz Dergisi, 29, ss.1 - 9, 2023. 10.4274/tod.galenos.2022.80148 |
ISNAD | tural, sengul vd. "Osteoporoz Epigenetiği". Türk Osteoporoz Dergisi 29/1 (2023), 1-9. https://doi.org/10.4274/tod.galenos.2022.80148 |