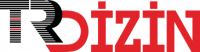
Hücrelerde Sıvı-Sıvı Faz Ayırımı ile Oluşan Membransız Cisimcikler: Literatür Derlemesi
Yıl: 2023 Cilt: 12 Sayı: 1 Sayfa Aralığı: 75 - 88 Metin Dili: Türkçe DOI: 10.5505/ktd.2022.56255 İndeks Tarihi: 03-06-2023
Hücrelerde Sıvı-Sıvı Faz Ayırımı ile Oluşan Membransız Cisimcikler: Literatür Derlemesi
Öz: Ökaryotik hücrelerde biyokimyasal süreçler organel adı verilen hücresel kompartmanlarda gerçekleşmektedir. Hücre içinde membranla çevrili organellerin yanı sıra membranla çevrelenmeyen yapılar da bulunmakta ve bu yapılar membransız cisimcikler olarak adlandırılmaktadır. Sıvı-sıvı faz ayırımı mekanizmasıyla oluşan bu cisimcikler, çekirdekte veya sitoplazmada oluşarak biyokimyasal süreçlerin hızlı ve verimli olarak gerçekleşmesini sağlamaktadır. Membransız cisimciklerde farklı hastalık gruplarında değişikliklerin saptanması nedeniyle, cisimciklerin yapı ve oluşumlarının anlaşılabilmesine yönelik araştırmalar son yıllarda hız kazanmıştır. Bu derlemede fizyolojik ve patolojik süreçlerde oluşan membransız cisimciklerin oluşum mekanizmaları, yapıları ve görevleri özetlenmiştir.
Anahtar Kelime: Membraneless Bodies of Cells Formed by Liquid-Liquid Phase Separation: Literature Review
Öz: Biochemical processes occur in cellular compartments, called organelles, in eukaryotic cells. In addition to membrane-enclosed organelles, non-membrane bound structures, namely membraneless bodies, are also found in cells. These bodies are formed in nucleus or cytoplasm by liquid-liquid phase separation mechanism and ensure that biochemical processes take place rapidly and efficiently. Since, alterations have been detected in membraneless bodies in different disease groups, investigations about the structure and formation of bodies have been accelerated in recent years. In this review, the mechanism of formation and structures of membraneless bodies were summarized in physiological and pathological states.
Anahtar Kelime: Belge Türü: Makale Makale Türü: Derleme Erişim Türü: Erişime Açık
- 1. Sirri V, Urcuqui-Inchima S, Roussel P ve Hernandez-Verdun D. Nucleolus: the fascinating nuclear body. Histochem Cell Biol 2008; 129, 13–31.
- 2. Cioce M ve Lamond A. Cajal bodies: A long history of discovery. Annu Rev Cell Dev Biol.2005; 21, 105-131.
- 3. Saunders T, Pan K, Angel A, Guan Y, Shah J, Howard M et.al. Noise reduction in the intracellular pom1p gradient by a dynamic clustering mechanism. Dev Cell. 2012; 22, 558-572.
- 4. Stoeger T, Battich N ve Pelkmans L. Passive noise filtering by cellular compartmentalization. Cell 2016; 164, 1151-1161.
- 5. Banani S, Lee H, Hyman A. veRosen M. Biomolecular condensates: organizers of cellular biochemistry. Nat Rev Mol Cell Biol 2017; 18, 285– 298.
- 6. Gomes E, Shorter J. The molecular language of membraneless organelles. J Biol Chem. 2019; 294 (18): 7115-7127.
- 7. Zhang H, Ji X, Li P, Liu C, Lou J, Wang Z et.al. Liquid-liquid phase separation in biology: mechanisms, physiological functions and human diseases. Sci China Life Sci. 2020; 63(7), 953-985.
- 8. Wang B, Zhang L, Dai T, Quin Z, Lu H, Zang L et.al. Liquid–liquid phase separation in human health and diseases. Sig Transduct Target Ther.2021;6, 290.
- 9. Mehta S ve Zhang J. Liquid–liquid phase separation drives cellular function and dysfunction in cancer. Nat Rev Cancer. 2022; 22, 239–252.
- 10. Mao Y, Zhang B ve Spector D. Biogenesis and function of nuclear bodies. Trends in genetics.2011; 27(8), 295–306.
- 11. Andersen J, Lam Y, Leung A, Ong S, Lyon C, Lamond A et.al. Nucleolar proteome dynamics. Nature. 2005; 433, 77–83.
- 12. Henderson A, Warburton D, Atwood K. Location of ribosomal DNA in the human chromosome complement. Proc Natl Acad Sci U S A. 1972;69(11):3394-3398.
- 13. Kalmárová M, Smirnov E, Masata M, Koberna K, Ligasová A, Popov A et.al. Positioning of NORs and NOR-bearing chromosomes in relation to nucleoli. J Struct Biol. 2007;160(1):49-56.
- 14. Boisvert F, van Koningsbruggen S, Navascués J, Lamond A. The multifunctional nucleolus. Nat Rev Mol Cell Biol. 2007;8(7):574-585.
- 15. Hadjiolov AA. The Nucleolus and Ribosome Biogenesis.Wien, NY: Springer Verlag; 1985.
- 16. M ́el`ese T, Xue Z. The nucleolus: an organelle formedby the act of building a ribosome. Curr Opin Cell Biol1995; 7:319–324
- 17. Pederson T. Cytologically "compact" nuclear domains. Nucleus 2010; 1:5, 444-445.
- 18. Pederson T. The plurifunctional nucleolus. Nucleic Acids Res. 1998;26(17):3871-3876.
- 19. Gerbi SA, Borovjagin AV, Lange TS. The nucleolus: A site of ribonucleoprotein maturation. Curr Opin Cell Biol. 2003; 15, 318325
- 20. Boulon S, Westman BJ, Hutten S, Boisvert FM, Lamond AI. The nucleolus under stress. Mol Cell. 2010;40(2):216-227.
- 21. Gebrane-Younes J, Sirri V, Junera H R, Roussel P, Hernandez-Verdun, D. Nucleolus: An essential nuclear domain. In Visions of the Cell Nucleus (Ed. Hemmerich P, veDiekmann, S.) 120-135, American Scientific Publishers, Valencia, CA, 2005
- 22. Tsai RY, Pederson T. Connecting the nucleolus to the cell cycle and human disease. FASEB J. 2014;28(8):3290-3296.
- 23. Hiscox JA. RNA viruses: hijacking the dynamic nucleolus. Nat Rev Microbiol. 2007;5(2):119-127.
- 24. Parlato R, Kreiner G. Nucleolar activity in neurodegenerative diseases: a missing piece of the puzzle?. J Mol Med (Berl). 2013;91(5):541-547.
- 25. Montanaro L, Treré D, Derenzini M. Nucleolus, ribosomes, and cancer. Am J Pathol. 2008;173(2):301- 310.
- 26. Matera AG, Shpargel KB. Pumping RNA: nuclear bodybuilding along the RNP pipeline. Curr Opin Cell Biol. 2006;18(3):317-324.
- 27. Gall JG. Cajal bodies: the first 100 years. Annu Rev Cell Dev Biol. 2000;16:273-300.
- 28. Matera AG, Terns RM, Terns MP. Non-coding RNAs: lessons from the small nuclear and small nucleolar RNAs. Nat Rev Mol Cell Biol. 2007;8(3):209-220.
- 29. Matera AG, Izaguire-Sierra M, Praveen K, Rajendra TK. Nuclear bodies: random aggregates of sticky proteins or crucibles of macromolecular assembly?. Dev Cell. 2009;17(5):639-647.
- 30. Dundr M. Nuclear bodies: multifunctional companions of the genome. Curr Opin Cell Biol. 2012;24(3):415-422.
- 31. Matera AG, Wang Z. A day in the life of the spliceosome. Nat Rev Mol Cell Biol. 2014;15(2):108- 121.
- 32. Darzacq X, Jády BE, Verheggen C, Kiss AM, Bertrand E, Kiss T. Cajal body-specific small nuclear RNAs: a novel class of 2'-O-methylation and pseudouridylation guide RNAs. EMBO J. 2002;21(11):2746-2756.
- 33. Jády BE, Bertrand E, Kiss T. Human telomerase RNA and box H/ACA scaRNAs share a common Cajal body-specific localization signal. J Cell Biol. 2004;164(5):647-652.
- 34. Zhu Y, Tomlinson RL, Lukowiak AA, Terns RM, Terns MP. Telomerase RNA accumulates in Cajal bodies in human cancer cells. Mol Biol Cell. 2004;15(1):81-90.
- 35. Woulfe J. Nuclear bodies in neurodegenerative disease. Biochim Biophys Acta. 2008;1783(11):2195- 2206.
- 36. Baltanás FC, Valero J, Alonso JR, Berciano MT, Lafarga M. Nuclear signs of pre-neurodegeneration. Methods Mol Biol. 2015;1254:43-54.
- 37. Tapia O, Bengoechea R, Palanca A, Arteaga R, Val-Bernal JF, Tizzano EF, Berciano MT, Lafarga M. Reorganization of Cajal bodies and nucleolar targeting of coilin in motor neurons of type I spinal muscular atrophy. Histochem Cell Biol. 2012;137(5):657-67
- 38. Liu Q, Dreyfuss G. A novel nuclear structure containing the survival of motor neurons protein. EMBO J. 1996;15(14):3555-3565.
- 39. Stanek D, Neugebauer KM. The Cajal body: a meeting place for spliceosomal snRNPs in the nuclear maze. Chromosoma. 2006;115(5):343-354.
- 40. Cauchi RJ. Gem depletion: amyotrophic lateral sclerosis and spinal muscular atrophy crossover. CNS Neurosci Ther. 2014;20(7):574-581.
- 41. DiDonato CJ, Parks RJ, Kothary R. Development of a gene therapy strategy for the restoration of survival motor neuron protein expression: implications for spinal muscular atrophy therapy. Hum Gene Ther. 2003;14(2):179-188.
- 42. Mattis VB, Rai R, Wang J, Chang CW, Coady T, Lorson CL. Novel aminoglycosides increase SMN levels in spinal muscular atrophy fibroblasts. Hum Genet. 2006;120(4):589-601.
- 43. Spector DL, Lamond AI. Nuclear speckles. Cold Spring Harb Perspect Biol. 2011;3(2):a000646.
- 44. Shopland LS, Johnson CV, Byron M, McNeil J, Lawrence JB. Clustering of multiple specific genes and gene-rich R-bands around SC-35 domains: evidence for local euchromatic neighborhoods. J Cell Biol. 2003;162(6):981-990.
- 45. Urbanek MO, Jazurek M, Switonski PM, Figura G, Krzyzosiak WJ. Nuclear speckles are detention centers for transcripts containing expanded CAG repeats. Biochim Biophys Acta. 2016;1862(9):1513- 1520.
- 46. Galganski L, Urbanek MO, Krzyzosiak WJ. Nuclear speckles: molecular organization, biological function and role in disease. Nucleic Acids Res. 2017;45(18):10350-10368.
- 47. Anczuków O, Krainer AR. Splicing-factor alterations in cancers. RNA. 2016;22(9):1285-1301.
- 48. Bond CS, Fox AH. Paraspeckles: nuclear bodies built on long noncoding RNA. J Cell Biol. 2009;186(5):637-644.
- 49. Chen LL, Carmichael GG. Altered nuclear retention of mRNAs containing inverted repeats in human embryonic stem cells: functional role of a nuclear noncoding RNA. Mol Cell. 2009;35(4):467- 478.
- 50. Clemson CM, Hutchinson JN, Sara SA, Ensminger A, Fox A, Chess A et.al. An architectural role for a nuclear noncoding RNA: NEAT1 RNA is essential for the structure of paraspeckles. Mol Cell. 2009;33(6):717-726.
- 51. Sunwoo H, Dinger ME, Wilusz JE, Amaral PP, Mattick JS, Spector DL. MEN epsilon/beta nuclear- retained non-coding RNAs are up-regulated upon muscle differentiation and are essential components of paraspeckles. Genome Res. 2009;19(3):347-359.
- 52. Sasaki YT, Ideue T, Sano M, Mituyama T, Hirose T. MENepsilon/beta noncoding RNAs are essential for structural integrity of nuclear paraspeckles. Proc Natl Acad Sci U S A. 2009;106(8):2525-2530.
- 53. Torres M, Becquet D, Blanchard MP, Guillen S, Boyer B, Moreno M et.al. Circadian RNA expression elicited by 3'-UTR IRAlu-paraspeckle associated elements. Elife. 2016;5:e14837.
- 54. Jiang L, Shao C, Wu QJ, Chen G, Zhou J, Yang B et.al. NEAT1 scaffolds RNA-binding proteins and the Microprocessor to globally enhance pri-miRNA processing. Nat Struct Mol Biol. 2017;24(10):816-824.
- 55. Zhang Z, Carmichael GG. The fate of dsRNA in the nucleus: a p54(nrb)-containing complex mediates the nuclear retention of promiscuously A-to-I edited RNAs. Cell. 2001;106(4):465-475
- 56. Zeng C, Liu S, Lu S, Yu X, Lai J, Wu Y et.al. The c-Myc-regulated lncRNA NEAT1 and paraspeckles modulate imatinib-induced apoptosis in CML cells. Mol Cancer. 2018;17(1):130.
- 57. Imamura K, Imamachi N, Akizuki G, Kumakura M, Kawaguchi A, Nagata K et.al. Long noncoding RNA NEAT1-dependent SFPQ relocation from promoter region to paraspeckle mediates IL8 expression upon immune stimuli. Mol Cell. 2014;53(3):393-406.
- 58. Zhang Q, Chen CY, Yedavalli VS, Jeang KT. NEAT1 long noncoding RNA and paraspeckle bodies modulate HIV-1 posttranscriptional expression. mBio. 2013;4(1):e00596-12.
- 59. Jin C, Peng X, Xie T, Lu X, Liu F, Wu H et.al. Detection of the long noncoding RNAs nuclear- enriched autosomal transcript 1 (NEAT1) and metastasis associated lung adenocarcinoma transcript 1 in the peripheral blood of HIV-1-infected patients. HIV Med. 2016;17(1):68-72.
- 60. Wang Z, Fan P, Zhao Y, Zhang S, Lu J, Xie W et.al. NEAT1 modulates herpes simplex virus-1 replication by regulating viral gene transcription. Cell Mol Life Sci. 2017;74(6):1117-1131.
- 61. Lallemand-Breitenbach V, de Thé H. PML nuclear bodies: from architecture to function. Curr Opin Cell Biol. 2018;52:154-161.
- 62. Van Damme E, Laukens K, Dang TH, Van Ostade X. A manually curated network of the PML nuclear body interactome reveals an important role for PML-NBs in SUMOylation dynamics. Int J Biol Sci. 2010;6(1):51-67.
- 63. Corpet A, Kleijwegt C, Roubille S,Juillard F, Jacquet K, Texier P et.al. PML nuclear bodies and chromatin dynamics: catch me if you can!. Nucleic Acids Res. 2020;48(21):11890-11912.
- 64. Boisvert FM, Hendzel MJ, Bazett-Jones DP. Promyelocytic leukemia (PML) nuclear bodies are protein structures that do not accumulate RNA. J Cell Biol. 2000;148(2):283-292.
- 65. Wang J, Shiels C, Sasieni P, Wu PJ, Islam SA, Freemont PS et.al. Promyelocytic leukemia nuclear bodies associate with transcriptionally active genomic regions. J Cell Biol. 2004;164(4):515-526.
- 66. Lehming N, Le Saux A, Schüller J, Ptashne M. Chromatin components as part of a putative transcriptional repressing complex. Proc Natl Acad Sci U S A. 1998;95(13):7322-7326.
- 67. Lallemand-Breitenbach V, de Thé H. PML nuclear bodies. Cold Spring Harb Perspect Biol. 2010;2(5):a000661.
- 68. Korb E, Finkbeiner S. PML in the Brain: From Development to Degeneration. Front Oncol. 2013;3:242.
- 69. Takahashi J, Fujigasaki H, Iwabuchi K, Bruni AC, Uchihara T, El Hachimi KH et.al. PML nuclear bodies and neuronal intranuclear inclusion in polyglutamine diseases. Neurobiol Dis. 2003;13(3):230-237.
- 70. Nizami Z, Deryusheva S, Gall JG. The Cajal body and histone locus body. Cold Spring Harb Perspect Biol. 2010;2(7):a000653.
- 71. Guglielmi B, La Rochelle N, Tjian R. Gene- specific transcriptional mechanisms at the histone gene cluster revealed by single-cell imaging. Mol Cell. 2013;51(4):480-492.
- 72. Tatomer DC, Terzo E, Curry KP, Salzleret H, Sabathal I, Zapotoczny G et.al Concentrating pre- mRNA processing factors in the histone locus body facilitates efficient histone mRNA biogenesis. J Cell Biol. 2016;213(5):557-570.
- 73. Sawyer IA, Dundr M. Nuclear bodies: Built to boost. J Cell Biol. 2016;213(5):509-511.
- 74. Bongiorno-Borbone L, De Cola A, Barcaroli D, Knight RA,Ilio CD, Melino G et.al. FLASH degradation in response to UV-C results in histone locus bodies disruption and cell-cycle arrest. Oncogene. 2010;29(6):802-810.
- 75. Tuckfield A, Clouston DR, Wilanowski TM, Zhao LL, Cunningham JM, Jane SM. Binding of the RING polycomb proteins to specific target genes in complex with the grainyhead-like family of developmental transcription factors. Mol Cell Biol. 2002;22(6):1936-1946.
- 76. Sawarkar R, Paro R. Interpretation of developmental signaling at chromatin: the Polycomb perspective. Dev Cell. 2010;19(5):651-661.
- 77. Hodgson JW, Brock HW. Are polycomb group bodies gene silencing factories?. Cell. 2011;144(2):170-171.
- 78. Liang Z, Biggin MD. Eve and ftz regulate a wide array of genes in blastoderm embryos: the selector homeoproteins directly or indirectly regulate most genes in Drosophila. Development. 1998;125(22):4471-4482.
- 79. Plath K, Talbot D, Hamer KM, Otte AP, Yang TP, Jaenischet R et.al. Developmentally regulated alterations in Polycomb repressive complex 1 proteins on the inactive X chromosome. J Cell Biol. 2004;167(6):1025-1035.
- 80. Sparmann A, van Lohuizen M. Polycomb silencers control cell fate, development and cancer. Nat Rev Cancer. 2006;6(11):846-856.
- 81. Villa R, Pasini D, Gutierrez A, Morey L, Occhionorelli M, Vire E, et al. Role of the polycomb repressive complex 2 in acute promyelocytic leukemia. Cancer Cell. 2007;11:513–525
- 82. Boukarabila H, Saurin AJ, Batsche E, Mossadegh N, van Lohuizen M, Otte AP, et al. The PRC1 Polycomb group complex interacts with PLZF/RARA to mediate leukemic transformation. Genes Dev. 2009;23:1195–1206.
- 83. Martinez AM, Cavalli G. The role of polycomb group proteins in cell cycle regulation during development. Cell Cycle. 2006;5(11):1189-1197.
- 84. Cao R, Wang L, Wang H, Xia L, Erdjument- Bromageet H, Tempst P et.al. Role of histone H3 lysine 27 methylation in Polycomb-group silencing. Science. 2002;298(5595):1039-1043.
- 85. Lee MG, Villa R, Trojer P, Norman J, Yan KP, Reinberg D et.al. Demethylation of H3K27 regulates polycomb recruitment and H2A ubiquitination. Science. 2007;318(5849):447-450.
- 86. Lund AH, van Lohuizen M. Polycomb complexes and silencing mechanisms. Curr Opin Cell Biol. 2004;16(3):239-246.
- 87. Ringrose L, Paro R. Epigenetic regulation of cellular memory by the Polycomb and Trithorax group proteins. Annu Rev Genet. 2004;38:413-443.
- 88. Wang W, Qin JJ, Voruganti S, Nag S, Zhou J, Zhang R. Polycomb Group (PcG) Proteins and Human Cancers: Multifaceted Functions and Therapeutic Implications. Med Res Rev. 2015;35(6):1220-1267.
- 89. Jolly C, Konecny L, Grady DL,Kutskova YA, Cotto JJ, Morimoto RI et.al. In vivo binding of active heat shock transcription factor 1 to human chromosome 9 heterochromatin during stress. J Cell Biol. 2002;156(5):775-781.
- 90. Jolly C, Metz A, Govin J, Vigneron M, Turner BM, Khochbin S et.al. Stress-induced transcription of satellite III repeats. J Cell Biol. 2004;164(1):25-33.
- 91. Biamonti G, Vourc'h C. Nuclear stress bodies. Cold Spring Harb Perspect Biol. 2010;2(6):a000695.
- 92. Collins M, Li Y, Bowser R. RBM45 associates with nuclear stress bodies and forms nuclear inclusions during chronic cellular stress and in neurodegenerative diseases. Acta Neuropathol Commun. 2020; 8, 91.
- 93. Huang S, Deerinck TJ, Ellisman MH, Spector DL. The dynamic organization of the perinucleolar compartment in the cell nucleus. J Cell Biol. 1997;137(5):965-974.
- 94. Matera AG, Frey MR, Margelot K, Wolin SL. A perinucleolar compartment contains several RNA polymerase III transcripts as well as the polypyrimidine tract-binding protein, hnRNP I. J Cell Biol. 1995;129(5):1181-1193.
- 95. Pollock C, Huang S. The perinucleolar compartment. Cold Spring Harb Perspect Biol. 2010;2(2):a000679.
- 96. Norton JT, Pollock CB, Wang C, Schink JC, Kim JJ, Huang S. Perinucleolar compartment prevalence is a phenotypic pancancer marker of malignancy. Cancer. 2008;113(4):861-869.
- 97. Carmo-Fonseca M, Berciano MT, Lafarga M. Orphan nuclear bodies. Cold Spring Harb Perspect Biol. 2010;2(9):a000703. doi:10.1101/cshperspect.a000703
- 98. Frisone P, Pradella D, Di Matteo A, Belloni E, Ghigna C, Paronetto MP. SAM68: Signal Transduction and RNA Metabolism in Human Cancer. Biomed Res Int. 2015;2015:528954.
- 99. Sánchez-Jiménez F, Sánchez-Margalet V. Role of Sam68 in post-transcriptional gene regulation. Int J Mol Sci. 2013;14(12):23402-23419.
- 100. Schultz LB, Chehab NH, Malikzay A, Halazonetis TD. p53 binding protein 1 (53BP1) is an early participant in the cellular response to DNA double-strand breaks. J Cell Biol. 2000;151(7):1381- 1390.
- 101. Lukas C, Savic V, Bekker-Jensen S, Doil C, Neumann B, Pedersen RS et.al. 53BP1 nuclear bodies form around DNA lesions generated by mitotic transmission of chromosomes under replication stress. Nat Cell Biol. 2011;13(3):243-253.
- 102. Harrigan JA, Belotserkovskaya R, Coates J, Dimitrova DS, Polo SE, Bradshaw CR et.al. Replication stress induces 53BP1-containing OPT domains in G1 cells. J Cell Biol. 2011;193(1):97-108.
- 103. Schmidt L, Wiedner M, Velimezi G, Prochazkova J, Owusuet M, Bauer S et.al. ATMIN is required for the ATM-mediated signaling and recruitment of 53BP1 to DNA damage sites upon replication stress. DNA Repair (Amst). 2014;24:122- 130.
- 104. Panier S, Boulton SJ. Double-strand break repair: 53BP1 comes into focus. Nat Rev Mol Cell Biol. 2014;15(1):7-18.
- 105. Hustedt N, Durocher D. The control of DNA repair by the cell cycle. Nat Cell Biol. 2016;19(1):1-9.
- 106. Lafarga M, Berciano MT, Pena E, Mayo I, Castaño JG, Bohmann D et.al. Clastosome: a subtype of nuclear body enriched in 19S and 20S proteasomes, ubiquitin, and protein substrates of proteasome. Mol Biol Cell. 2002;13(8):2771-2782.
- 107. Schul W, Groenhout B, Koberna K, Takagaki Y, Jenny A, Manders EM et.al. The RNA 3' cleavage factors CstF 64 kDa and CPSF 100 kDa are concentrated in nuclear domains closely associated with coiled bodies and newly synthesized RNA. EMBO J. 1996;15(11):2883-2892.
- 108. Li L, Roy K, Katyal S, Sun X, Bléoo S, Godbout R. Dynamic nature of cleavage bodies and their spatial relationship to DDX1 bodies, Cajal bodies, and gems. Mol Biol Cell. 2006;17(3):1126-1140.
- 109. Pombo A, Cuello P, Schul W, Yoon JB, Roeder RG, Cooket PB et.al. Regional and temporal specialization in the nucleus: a transcriptionally-active nuclear domain rich in PTF, Oct1 and PIKA antigens associates with specific chromosomes early in the cell cycle. EMBO J. 1998;17(6):1768-1778.
- 110. Navascués J, Bengoechea R, Tapia O, Vaqué JP, Lafarga M, Berciano MT. Characterization of a new SUMO-1 nuclear body (SNB) enriched in pCREB, CBP, c-Jun in neuron-like UR61 cells. Chromosoma. 2007;116(5):441-451.
- 111. Eulalio A, Behm-Ansmant I, Izaurralde E. P bodies: at the crossroads of post-transcriptional pathways. Nat Rev Mol Cell Biol. 2007;8(1):9-22.
- 112. Standart N. Weil D. P-Bodies: Cytosolic Droplets for Coordinated mRNA Storage. Trends Genet. 2018, 34,612–626.
- 113. Tian S, Curnutte HA, Trcek T. RNA Granules: A View from the RNA Perspective. Molecules. 2020 Jul 8;25(14):3130.
- 114. Horvathova I, Voigt F, Kotrys AV,Zhan Y, Artus-Revel CG, Eglingeret J et.al The Dynamics of mRNA Turnover Revealed by Single-Molecule Imaging in Single Cells. Mol Cell. 2017;68(3):615- 625.e9.
- 115. Riggs CL, Kedersha N, Ivanov P, Anderson P. Mammalian stress granules and P bodies at a glance. J Cell Sci. 2020;133(16):jcs242487.
- 116. Liu JL, Gall JG. U bodies are cytoplasmic structures that contain uridine-rich small nuclear ribonucleoproteins and associate with P bodies. Proc Natl Acad Sci U S A. 2007;104(28):11655-11659.
- 117. Moser JJ, Fritzler MJ. Cytoplasmic ribonucleoprotein (RNP) bodies and their relationship to GW/P bodies. Int J Biochem Cell Biol. 2010;42(6):828-843.
- 118. Moujaber O, Stochaj U. Cytoplasmic RNA Granules in Somatic Maintenance. Gerontology. 2018;64(5):485-494.
- 119. Buckingham M, Liu JL. U bodies respond to nutrient stress in Drosophila. Exp Cell Res. 2011;317(20):2835-2844.
- 120. Kedersha N, Anderson P. Mammalian stress granules and processing bodies. Methods Enzymol. 2007;431:61-81.
- 121. Kedersha NL, Gupta M, Li W, Miller I, Anderson P. RNA-binding proteins TIA-1 and TIAR link the phosphorylation of eIF-2 alpha to the assembly of mammalian stress granules. J Cell Biol. 1999;147(7):1431-1442.
- 122. Vanderweyde T, Youmans K, Liu-Yesucevitz L, Wolozin B. Role of stress granules and RNA-binding proteins in neurodegeneration: a mini-review. Gerontology. 2013;59(6):524-533.
- 123. Anderson P, Kedersha N. Stress granules. Curr Biol. 2009;19(10):R397-R398.
- 124. Buchan JR, Parker R. Eukaryotic stress granules: the ins and outs of translation. Mol Cell. 2009;36(6):932-941.
- 125. Parker R, Sheth U. P bodies and the control of mRNA translation and degradation. Mol Cell. 2007;25(5):635-646.
- 126. Kedersha N, Anderson P. Stress granules: sites of mRNA triage that regulate mRNA stability and translatability. Biochem Soc Trans. 2002;30(Pt 6):963- 969.
- 127. Onomoto K, Yoneyama M, Fung G, Kato H, Fujita T. Antiviral innate immunity and stress granule responses. Trends Immunol. 2014;35(9):420-428.
- 128. Song MS, Grabocka E. Stress Granules in Cancer. Rev Physiol Biochem Pharmacol. 2020;10.1007/112_2020_37.
- 129. Asadi MR, Sadat Moslehian M, Sabaie H, Jalaiei A, Ghafouri-Fard S, Taheriet M et al. Stress Granules and Neurodegenerative Disorders: A Scoping Review. Front Aging Neurosci. 2021;13:650740.
- 130. Wolozin B, Ivanov P. Stress granules and neurodegeneration. Nat Rev Neurosci. 2019;20(11):649-666.
- 131. Mateju D, Franzmann TM, Patel A,Kopach A, Boczek EE, Maharana S et.al. An aberrant phase transition of stress granules triggered by misfolded protein and prevented by chaperone function. EMBO J. 2017;36(12):1669-1687.
- 132. St Johnston D. Moving messages: the intracellular localization of mRNAs. Nat Rev Mol Cell Biol. 2005;6(5):363-375.
- 133. Hillebrand J, Barbee SA, Ramaswami M. P-body components, microRNA regulation, and synaptic plasticity. ScientificWorldJournal. 2007;7:178-190.
- 134. Anderson P, Kedersha N. RNA granules. J Cell Biol. 2006;172(6):803-808.
- 135. Cougot N, Bhattacharyya SN, Tapia-Arancibia L,Bordonné R, Filipowicz W, Bertrandet E et.al. Dendrites of mammalian neurons contain specialized P-body-like structures that respond to neuronal activation. J Neurosci. 2008;28(51):13793-13804.
- 136. Zeitelhofer M, Karra D, Macchi P, Tolino M, Thomas S, Schwarzet M et al. Dynamic interaction between P-bodies and transport ribonucleoprotein particles in dendrites of mature hippocampal neurons. J Neurosci. 2008;28(30):7555-7562.
- 137. Jønson L, Vikesaa J, Krogh A, et al. Molecular composition of IMP1 ribonucleoprotein granules. Mol Cell Proteomics. 2007;6(5):798-811.
- 138. Moschner K, Sündermann F, Meyer H, Pereira da Graca A, Appel N, Paululatet A et.al. RNA protein granules modulate tau isoform expression and induce neuronal sprouting. J Biol Chem. 2014;289(24):16814- 16825.
- 139. Kourtidis A, Necela B, Lin WH,Lu R, Feathers RW, Asmann YW et.al. Cadherin complexes recruit mRNAs and RISC to regulate epithelial cell signaling. J Cell Biol. 2017;216(10):3073-3085.
- 140. Jourdain AA, Boehm E, Maundrell K, Martinou JC. Mitochondrial RNA granules: Compartmentalizing mitochondrial gene expression. J Cell Biol. 2016;212(6):611-614.
- 141. RaffJW. Phase Separation and the Centrosome: A Fait Accompli?. Trends Cell Biol. 2019;29(8):612-622.
- 142. Odabasi E, Batman U, Firat-Karalar EN. Unraveling the mysteries of centriolar satellites: time to rewrite the textbooks about the centrosome/cilium complex. Mol Biol Cell. 2020;31(9):866-872.
- 143. Odabasi E, Gul S, Kavakli IH, Firat-Karalar EN. Centriolar satellites are required for efficient ciliogenesis and ciliary content regulation. EMBO Rep. 2019;20(6):e47723.
- 144. Voronina E, Seydoux G, Sassone-Corsi P, Nagamori I. RNA granules in germ cells. Cold Spring Harb Perspect Biol. 2011;3(12):a002774.
- 145. Mukherjee N, Mukherjee C. Germ cell ribonucleoprotein granules in different clades of life: From insects to mammals. Wiley Interdiscip Rev RNA. 2021;12(4):e1642.
- 146. Ross CA, Poirier MA. Protein aggregation and neurodegenerative disease. Nat Med. 2004;10 Suppl:S10-S17.
- 147. Bersuker K, Brandeis M, Kopito RR. Protein misfolding specifies recruitment to cytoplasmic inclusion bodies. J Cell Biol. 2016;213(2):229-241.
- 148. Arrasate M, Mitra S, Schweitzer ES, Segal MR, Finkbeiner S. Inclusion body formation reduces levels of mutant huntingtin and the risk of neuronal death. Nature. 2004;431(7010):805-810.
- 149. Luo Y, Na Z, Slavoff SA. P-Bodies: Composition, Properties, and Functions. Biochemistry. 2018;57(17):2424-2431
- 150. Rey T, Zaganelli S, Cuillery E, Vartholomaiou E, Croisier M, Martinou JC et.al. Mitochondrial RNA granules are fluid condensates positioned by membrane dynamics. Nat Cell Biol. 2020;22(10):1180-1186.
- 151. Jourdain AA, Koppen M, Wydro M,Rodley CD, Lightowlers RN, Chrzanowska-LightowlersZM et.al. GRSF1 regulates RNA processing in mitochondrial RNA granules. Cell Metab. 2013;17(3):399-410.
- 152. Meikar O, Vagin VV, Chalmel F, Sõstar K, Lardenois A, Hammell M et.al. An atlas of chromatoid body components. RNA. 2014;20(4):483-495.
- 153. Marlow FL. Maternal Control of Development in Vertebrates: My Mother Made Me Do It!. San Rafael (CA): Morgan & Claypool Life Sciences; 2010.
- 154. Morroni M, Cangiotti AM, Marzioni D, D'Angelo A, Gesuita R, De Nictolis M. Intermitochondrial cement (nuage) in a spermatocytic seminoma: comparison with classical seminoma and normal testis. Virchows Arch. 2008;453(2):189-196.
APA | GUMUS D, BORA G (2023). Hücrelerde Sıvı-Sıvı Faz Ayırımı ile Oluşan Membransız Cisimcikler: Literatür Derlemesi. , 75 - 88. 10.5505/ktd.2022.56255 |
Chicago | GUMUS Dudu,BORA Gamze Hücrelerde Sıvı-Sıvı Faz Ayırımı ile Oluşan Membransız Cisimcikler: Literatür Derlemesi. (2023): 75 - 88. 10.5505/ktd.2022.56255 |
MLA | GUMUS Dudu,BORA Gamze Hücrelerde Sıvı-Sıvı Faz Ayırımı ile Oluşan Membransız Cisimcikler: Literatür Derlemesi. , 2023, ss.75 - 88. 10.5505/ktd.2022.56255 |
AMA | GUMUS D,BORA G Hücrelerde Sıvı-Sıvı Faz Ayırımı ile Oluşan Membransız Cisimcikler: Literatür Derlemesi. . 2023; 75 - 88. 10.5505/ktd.2022.56255 |
Vancouver | GUMUS D,BORA G Hücrelerde Sıvı-Sıvı Faz Ayırımı ile Oluşan Membransız Cisimcikler: Literatür Derlemesi. . 2023; 75 - 88. 10.5505/ktd.2022.56255 |
IEEE | GUMUS D,BORA G "Hücrelerde Sıvı-Sıvı Faz Ayırımı ile Oluşan Membransız Cisimcikler: Literatür Derlemesi." , ss.75 - 88, 2023. 10.5505/ktd.2022.56255 |
ISNAD | GUMUS, Dudu - BORA, Gamze. "Hücrelerde Sıvı-Sıvı Faz Ayırımı ile Oluşan Membransız Cisimcikler: Literatür Derlemesi". (2023), 75-88. https://doi.org/10.5505/ktd.2022.56255 |
APA | GUMUS D, BORA G (2023). Hücrelerde Sıvı-Sıvı Faz Ayırımı ile Oluşan Membransız Cisimcikler: Literatür Derlemesi. Kocaeli Tıp Dergisi, 12(1), 75 - 88. 10.5505/ktd.2022.56255 |
Chicago | GUMUS Dudu,BORA Gamze Hücrelerde Sıvı-Sıvı Faz Ayırımı ile Oluşan Membransız Cisimcikler: Literatür Derlemesi. Kocaeli Tıp Dergisi 12, no.1 (2023): 75 - 88. 10.5505/ktd.2022.56255 |
MLA | GUMUS Dudu,BORA Gamze Hücrelerde Sıvı-Sıvı Faz Ayırımı ile Oluşan Membransız Cisimcikler: Literatür Derlemesi. Kocaeli Tıp Dergisi, vol.12, no.1, 2023, ss.75 - 88. 10.5505/ktd.2022.56255 |
AMA | GUMUS D,BORA G Hücrelerde Sıvı-Sıvı Faz Ayırımı ile Oluşan Membransız Cisimcikler: Literatür Derlemesi. Kocaeli Tıp Dergisi. 2023; 12(1): 75 - 88. 10.5505/ktd.2022.56255 |
Vancouver | GUMUS D,BORA G Hücrelerde Sıvı-Sıvı Faz Ayırımı ile Oluşan Membransız Cisimcikler: Literatür Derlemesi. Kocaeli Tıp Dergisi. 2023; 12(1): 75 - 88. 10.5505/ktd.2022.56255 |
IEEE | GUMUS D,BORA G "Hücrelerde Sıvı-Sıvı Faz Ayırımı ile Oluşan Membransız Cisimcikler: Literatür Derlemesi." Kocaeli Tıp Dergisi, 12, ss.75 - 88, 2023. 10.5505/ktd.2022.56255 |
ISNAD | GUMUS, Dudu - BORA, Gamze. "Hücrelerde Sıvı-Sıvı Faz Ayırımı ile Oluşan Membransız Cisimcikler: Literatür Derlemesi". Kocaeli Tıp Dergisi 12/1 (2023), 75-88. https://doi.org/10.5505/ktd.2022.56255 |