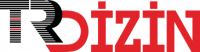
Epigenetic Code for Cell Fate During Development and Disease in Human
Yıl: 2023 Cilt: 7 Sayı: 2 Sayfa Aralığı: 95 - 102 Metin Dili: İngilizce DOI: 10.14744/ejmo.2023.42324 İndeks Tarihi: 05-07-2023
Epigenetic Code for Cell Fate During Development and Disease in Human
Öz: Epigenetic reprogramming is the leading mechanism for cell differentiation in early development which gradually takes place upon zygote formation. This is governed by epigenetic modifications of genes involved in cell differen- tiation defined by Waddington’s landscape. Somatic cells have specific gene expression profiles regulated by distinct epigenetic patterns. Therefore, they maintain their identity and specific gene profiles throughout lifetime. Although somatic cells can be induced into stem cell-like structures, the possible transformation of the cells can be associated with disruptions in cell identity leading to carcinogenesis. The epigenetic code for cell identity is the crucial player for maintaining stability and wellness of the cells during their lifespan. This review summarizes the epigenetic regulations involved in establishment of cellular fate and their abnormalities in cancer.
Anahtar Kelime: Belge Türü: Makale Makale Türü: Derleme Erişim Türü: Erişime Açık
- 1. Huntriss J. Epigenetic reprogramming in the embryo. Epi- genetics Reprod Heal 2021:97–116.
- 2. Niemann H. Epigenetic reprogramming in mammalian spe - cies after SCNT-based cloning. Theriogenology 2016;86:80– 90.
- 3. Ogura A, Matoba S, Inoue K. 25th anniversary of cloning by somatic-cell nuclear transfer: Epigenetic abnormalities as- sociated with somatic cell nuclear transfer. Reproduction 2021;162:F45–F58.
- 4. Dean W, Santos F, Stojkovic M, Zakhartchenko V, Walter J, Wolf E, et al. Conservation of methylation reprogramming in mammalian development: aberrant reprogramming in cloned em- bryos. Proc Natl Acad Sci U S A 2001;98:13734–8.
- 5. Cao P, Li H, Zuo Y, Nashun B. Characterization of DNA meth- ylation patterns and mining of epigenetic markers during ge- nomic reprogramming in SCNT embryos. Front Cell Dev Biol 2020;8:570107.
- 6. Fujii K, Tanaka S, Hasegawa T, Narazaki M, Kumanogoh A, Koseki H, et al. Tet DNA demethylase is required for plasma cell differentiation by controlling expression levels of IRF4. Int Immunol 2020;32:683–90.
- 7. Izzo F, Lee SC, Poran A, Chaligne R, Gaiti F, Gross B, et al. DNA methylation disruption reshapes the hematopoietic differen- tiation landscape. Nat Genet. 2020;52:378–87.
- 8. Gehre M, Bunina D, Sidoli S, Lübke MJ, Diaz N, Trovato M, et al. Lysine 4 of histone H3.3 is required for embryonic stem cell differentiation, histone enrichment at regulatory regions and transcription accuracy. Nat Genet 2020;52:273–282.
- 9. Abe S, Nagatomo H, Sasaki H, Ishiuchi T. A histone H3.3K36M mutation in mice causes an imbalance of histone modifica- tions and defects in chondrocyte differentiation. Epigenetics 2021;16:1123–34.
- 10. Aloia L. Epigenetic regulation of cell-fate changes that deter- mine adult liver regeneration after injury. Front Cell Dev Biol 2021;9:346.
- 11. Lister R, Pelizzola M, Dowen RH, Hawkins RD, Hon G, Tonti-Filip- pini J, et al. Human DNA methylomes at base resolution show widespread epigenomic differences. Nature 2009;462:315–22
- 12. Varley KE, Gertz J, Bowling KM, Parker SL, Reddy TE, Pauli-Behn F, et al. Dynamic DNA methylation across diverse human cell lines and tissues. Genome Res 2013;23:555–67.
- 13. Yang X, Han H, De Carvalho DD, Lay FD, Jones PA, Liang G. Gene body methylation can alter gene expression and is a therapeutic target in cancer. Cancer Cell 2014;26:577–90.
- 14. Laurent L, Wong E, Li G, Huynh T, Tsirigos A, Ong CT, et al. Dy- namic changes in the human methylome during differentia- tion. Genome Res 2010;20:320–31.
- 15. Bannister AJ, Kouzarides T. Regulation of chromatin by his- tone modifications. Cell Res 2011;21:381–95.
- 16. Choudhary C, Kumar C, Gnad F, Nielsen ML, Rehman M, Wal- ther TC, et al. Lysine acetylation targets protein complexes and co-regulates major cellular functions. Science 2009;325:834– 40.
- 17. Zhang B, Gu X, Han X, Gao Q, Liu J, Guo T, et al. Crosstalk between DNA methylation and histone acetylation trig- gers GDNF high transcription in glioblastoma cells. Clin Epi- genetics 2020;12:47.
- 18. Fu K, Bonora G, Pellegrini M. Interactions between core his- tone marks and DNA methyltransferases predict DNA meth- ylation patterns observed in human cells and tissues. Epi- genetics 2020;15:272–82.
- 19. Santini L, Halbritter F, Titz-Teixeira F, Suzuki T, Asami M, Ma X, et al. Genomic imprinting in mouse blastocysts is predominantly associated with H3K27me3. Nat Commun 2021;12:3804.
- 20. Chang S, Wang Y, Xin Y, Wang S, Luo Y, Wang L, et al. DNA methylation abnormalities of imprinted genes in congenital heart disease: a pilot study. BMC Med Genomics 2021;14:4.
- 21. Elbracht M, Mackay D, Begemann M, Kagan KO, Eggermann T. Disturbed genomic imprinting and its relevance for human reproduction: Causes and clinical consequences. Hum Reprod Update 2020;26:197–213.
- 22. Pastor WA, Aravind L, Rao A. TETonic shift: Biological roles of TET proteins in DNA demethylation and transcription. Nat Rev Mol Cell Biol 2013;14:341–56.
- 23. Vallot C, Ouimette JF, Rougeulle C. Establishment of X chro- mosome inactivation and epigenomic features of the inactive X depend on cellular contexts. BioEssays 2016;38:869–80.
- 24. Hasegawa Y, Taylor D, Ovchinnikov DA, Wolvetang EJ, de Tor- renté L, Mar JC. Variability of gene expression identifies tran- scriptional regulators of early human embryonic develop- ment. PLOS Genet 2015;11:e1005428.
- 25. Burton A, Brochard V, Galan C, Ruiz-Morales ER, Rovira Q, Ter- rones DR, et al. Heterochromatin establishment during early mammalian development is regulated by pericentromeric RNA and characterized by non-repressive H3K9me3. Nat Cell Biol 2020;22:767–78.
- 26. Waddington C. How do cells differentiate? Sci Am 1953;189:108–17.
- 27. Shock T, Badang L, Ferguson B, Martinez-Guryn K. The inter- play between diet, gut microbes, and host epigenetics in health and disease. J Nutr Biochem 2021;95:108631.
- 28. Gomez-Verjan JC, Esparza-Aguilar M, Martín-Martín V, Salazar- Perez C, Cadena-Trejo C, Gutierrez-Robledo LM, et al. Years of schooling could reduce epigenetic aging: a study of a Mexi- can cohort. Genes (Basel) 2021;12:1408.
- 29. Fiorito G, McCrory C, Robinson O, Carmeli C, Ochoa-Rosales C, Zhang Y, et al; BIOS Consortium; Lifepath consortium. So- cioeconomic position, lifestyle habits and biomarkers of epi- genetic aging: a multi-cohort analysis. Aging (Albany NY) 2019;11:2045–70.
- 30. Perna L, Zhang Y, Matias-Garcia PR, Ladwig KH, Wiechmann T, Wild B, et al. Subjective mental health, incidence of depres- sive symptoms in later life, and the role of epigenetics: re- sults from two longitudinal cohort studies. Transl Psychiatry 2020;10:323.
- 31. Venditti S, Verdone L, Reale A, Vetriani V, Caserta M, Zampieri M. Molecules of silence: effects of meditation on gene expres- sion and epigenetics. Front Psychol 2020;11:1767.
- 32. Keshawarz A, Joehanes R, Guan W, Huan T, DeMeo DL, Grove ML, et al. Longitudinal change in blood DNA epigenetic signa- ture after smoking cessation. Epigenetics 2022;17:1098–109.
- 33. Lundberg E, Fagerberg L, Klevebring D, Matic I, Geiger T, Cox J, et al. Defining the transcriptome and proteome in three functionally different human cell lines. Mol Syst Biol 2010;6:450.
- 34. Kingston RE, Tamkun JW. Transcriptional regulation by trithorax-group proteins. Cold Spring Harb Perspect Biol 2014;6:a019349.
- 35. Francis NJ, Kingston RE. Mechanisms of transcriptional mem- ory. Nat Rev Mol Cell Biol 2001;2:409–21.
- 36. Lefrançois L, Obar JJ. Once a killer, always a killer: From cyto- toxic T cell to memory cell. Immunol Rev 2010;235:206–18.
- 37. Kaufman J, Graf BA, Leung EC, Pollock SJ, Koumas L, Reddy SY, et al. Fibroblasts as sentinel cells: role of the CDcd40-CDcd40 ligand system in fibroblast activation and lung inflammation and fibrosis. Chest 2001;120:53–5.
- 38. Hamada A, Torre C, Drancourt M, Ghigo E. Trained immunity carried by non-immune cells. Front Microbiol 2019;9:3225.
- 39. Bustos-Arriaga J, García-Machorro J, León-Juárez M, García- Cordero J, Santos-Argumedo L, Flores-Romo L, et al. Activa- tion of the innate immune response against DENV in nor - mal non-transformed human fibroblasts. PLoS Negl Trop Dis 2011;5:e1420.
- 40. Kim M, Costello J. DNA methylation: An epigenetic mark of cellular memory. Exp Mol Med 2017;49:e322.
- 41. Henikoff S, Greally JM. Epigenetics, cellular memory and gene regulation. Curr Biol 2016;26:R644–8.
- 42. Ringrose L, Paro R. Epigenetic regulation of cellular memory by the polycomb and trithorax group proteins. Annu Rev Gen- et 2004;38:413–43.
- 43. Yin Y, Morgunova E, Jolma A, Kaasinen E, Sahu B, Khund- Sayeed S, et al. Impact of cytosine methylation on DNA binding specificities of human transcription factors. Science 2017;356:eaaj2239.
- 44. Reizel Y, Morgan A, Gao L, Schug J, Mukherjee S, García MF, et al. FoxA-dependent demethylation of DNA initiates epigen- etic memory of cellular identity. Dev Cell 2021;56:602–12.e4.
- 45. Wen Y, Dungan CM, Mobley CB, Valentino T, von Walden F, Murach KA. Nucleus type-specific DNA methylomics reveals epigenetic "memory" of prior adaptation in skeletal muscle. Function (Oxf ) 2021;2:zqab038.
- 46. Vierbuchen T, Ostermeier A, Pang ZP, Kokubu Y, Südhof TC, Wernig M. Direct conversion of fibroblasts to functional neu- rons by defined factors. Nature 2010;463:1035–41.
- 47. Matsuda T, Irie T, Katsurabayashi S, Hayashi Y, Nagai T, Hamaza- ki N, et al. Pioneer factor NeuroD1 rearranges transcriptional and epigenetic profiles to execute microglia-neuron conver- sion. Neuron 2019;101:472–85.e7.
- 48. Chen L, Ge B, Casale FP, Vasquez L, Kwan T, Garrido-Martín D, et al. Genetic drivers of epigenetic and transcriptional varia- tion in human immune cells. Cell 2016;167:1398–414.e24.
- 49. Roy R, Ramamoorthy S, Shapiro BD, Kaileh M, Hernandez D, Sa- rantopoulou D, et al. DNA methylation signatures reveal that distinct combinations of transcription factors specify human im- mune cell epigenetic identity. Immunity 2021;54:2465–80.e5.
- 50. Gutierrez-Arcelus M, Ongen H, Lappalainen T, Montgomery SB, Buil A, Yurovsky A, et al. Tissue-specific effects of genetic and epigenetic variation on gene regulation and splicing. PLoS Genet 2015;11:e1004958.
- 51. Oh C, Kim HR, Oh S, Ko JY, Kim Y, Kang K, et al. Epigenetic up- regulation of MAGE-A isoforms promotes breast cancer cell aggressiveness. Cancers (Basel) 2021;13:3176.
- 52. Berry K, Wang J, Lu QR. Epigenetic regulation of oligodendro- cyte myelination in developmental disorders and neurode- generative diseases. F1000Res 2020;9:F1000 Faculty Rev–105.
- 53. Ohkura N, Sakaguchi S. Transcriptional and epigenetic basis of Treg cell development and function: its genetic anomalies or variations in autoimmune diseases. Cell Res 2020;30:465– 74.
- 54. Wu X, Cheng YL, Matthen M, Yoon A, Schwartz GK, Bala S, et al. Down-regulation of the tumor suppressor miR-34a con- tributes to head and neck cancer by up-regulating the MET oncogene and modulating tumor immune evasion. J Exp Clin Cancer Res 2021;40:70.
- 55. Xing H, Wang P, Liu S, Jing S, Lin J, Yang J, et al. A global in- tegrated analysis of UNC5C down-regulation in cancers: in- sights from mechanism and combined treatment strategy. Biomed Pharmacother 2021;138:111355.
- 56. Tokay E. Epidermal growth factor mediates up-regulation of URGCP oncogene in human hepatoma cancer cells. Mol Biol 2021;55:618–23.
- 57. Zhang W, Klinkebiel D, Barger CJ, Pandey S, Guda C, Miller A, et al. Global DNA hypomethylation in epithelial ovarian cancer: passive demethylation and association with genomic instabil- ity. Cancers (Basel) 2020;12:764.
- 58. Cerapio JP, Marchio A, Cano L, López I, Fournié JJ, Régnault B, et al. Global DNA hypermethylation pattern and unique gene expression signature in liver cancer from patients with Indig- enous American ancestry. Oncotarget 2021;12:475–92.
- 59. Pfeifer GP. Defining driver DNA methylation changes in hu- man cancer. Int J Mol Sci 2018;19:1166.
- 60. Danilova L, Wrangle J, Herman JG, Cope L. DNA-methylation for the detection and distinction of 19 human malignancies. Epigenetics 2022;17:191–201.
- 61. Smith AR, Smith RG, Burrage J, Troakes C, Al-Sarraj S, Kalaria RN, et al. A cross-brain regions study of ANK1 DNA methyla- tion in different neurodegenerative diseases. Neurobiol Aging 2019;74:70–6.
- 62. O’Neill KM, Irwin RE, Mackin SJ, Thursby SJ, Thakur A, Bertens C, et al. Depletion of DNMT1 in differentiated human cells highlights key classes of sensitive genes and an interplay with polycomb repression. Epigenetics and Chromatin 2018;11:12.
- 63. Biniszkiewicz D, Gribnau J, Ramsahoye B, Gaudet F, Eggan K, Humpherys D, et al. Dnmt1 overexpression causes genomic hypermethylation, loss of imprinting, and embryonic lethal- ity. Mol Cell Biol 2002;22:2124–35.
- 64. Alaterre E, Ovejero S, Herviou L, de Boussac H, Papadopou- los G, Kulis M, et al. Comprehensive characterization of the epigenetic landscape in Multiple Myeloma. Theranostics 2022;12:1715–29.
- 65. Peedicayil J. The potential role of epigenetic drugs in the treatment of anxiety disorders. Neuropsychiatr Dis Treat 2020;16:597–606.
- 66. Montalvo-Casimiro M, González-Barrios R, Meraz-Rodriguez MA, Juárez-González VT, Arriaga-Canon C, Herrera LA. Epidrug repurposing: discovering new faces of old acquaintances in cancer therapy. Front Oncol 2020;10:605386.
- 67. Megiorni F, Camero S, Pontecorvi P, Camicia L, Marampon F, Ceccarelli S, et al. OTX015 epi-drug exerts antitumor effects in ovarian cancer cells by blocking GNL3-mediated radiore- sistance mechanisms: cellular, molecular and computational evidence. Cancers (Basel) 2021;13:1519.
- 68. Shi F, Li Y, Han R, Fu A, Wang R, Nusbaum O, et al. Valerian and valeric acid inhibit growth of breast cancer cells possibly by mediating epigenetic modifications. Sci Rep 2021;11:2519
- 69. Brodská B, Holoubek A, Otevřelová P, Kuželová K. Com- bined treatment with low concentrations of decitabine and SAHA causes cell death in leukemic cell lines but not in normal peripheral blood lymphocytes. Biomed Res Int 2013;2013:659254.
- 70. Li X, Zhang M, Cai S, Wu Y, You Y, Wang X, et al. Concentration- dependent decitabine effects on primary NK cells viability, phenotype, and function in the absence of obvious NK cells proliferation-original article. Front Pharmacol 2021;12:755662.
- 71. Papulino C, Chianese U, Nicoletti MM, Benedetti R, Altucci L. Preclinical and clinical epigenetic-based reconsideration of beckwith-wiedemann syndrome. Front Genet 2020;11:1112.
- 72. Lu W, Zhang R, Jiang H, Zhang H, Luo C. Computer-aided drug design in epigenetics. Front Chem 2018 ;6:57.
- 73. Feinberg AP, Koldobskiy MA, Göndör A. Epigenetic modula- tors, modifiers and mediators in cancer aetiology and pro- gression. Nat Rev Genet 2016;17:284–99.
- 74. Mohammad HP, Barbash O, Creasy CL. Targeting epigenetic modifications in cancer therapy: erasing the roadmap to can- cer. Nat Med 2019;25:403–18.
- 75. Cheng H, Zou Y, Shah CD, Fan N, Bhagat TD, Gucalp R, et al. First-in-human study of inhaled Azacitidine in patients with advanced non-small cell lung cancer. Lung Cancer 2021;154:99–104.
- 76. Huls G, Chitu DA, Havelange V, Jongen-Lavrencic M, van de Loosdrecht AA, Biemond BJ, et al; Dutch-Belgian Hemato- Oncology Cooperative Group (HOVON). Azacitidine mainte- nance after intensive chemotherapy improves DFS in older AML patients. Blood 2019;133:1457–64.
- 77. Sapozhnikov DM, Szyf M. Unraveling the functional role of DNA demethylation at specific promoters by targeted steric blockage of DNA methyltransferase with CRISPR/dCas9. Nat Commun 2021;12:5711.
- 78. Shoaib M, Chen Q, Shi X, Nair N, Prasanna C, Yang R, et al. His- tone H4 lysine 20 mono-methylation directly facilitates chro- matin openness and promotes transcription of housekeeping genes. Nat Commun 2021;12:4800.
APA | Celik S (2023). Epigenetic Code for Cell Fate During Development and Disease in Human. , 95 - 102. 10.14744/ejmo.2023.42324 |
Chicago | Celik Selcen Epigenetic Code for Cell Fate During Development and Disease in Human. (2023): 95 - 102. 10.14744/ejmo.2023.42324 |
MLA | Celik Selcen Epigenetic Code for Cell Fate During Development and Disease in Human. , 2023, ss.95 - 102. 10.14744/ejmo.2023.42324 |
AMA | Celik S Epigenetic Code for Cell Fate During Development and Disease in Human. . 2023; 95 - 102. 10.14744/ejmo.2023.42324 |
Vancouver | Celik S Epigenetic Code for Cell Fate During Development and Disease in Human. . 2023; 95 - 102. 10.14744/ejmo.2023.42324 |
IEEE | Celik S "Epigenetic Code for Cell Fate During Development and Disease in Human." , ss.95 - 102, 2023. 10.14744/ejmo.2023.42324 |
ISNAD | Celik, Selcen. "Epigenetic Code for Cell Fate During Development and Disease in Human". (2023), 95-102. https://doi.org/10.14744/ejmo.2023.42324 |
APA | Celik S (2023). Epigenetic Code for Cell Fate During Development and Disease in Human. Eurasian Journal of Medicine and Oncology, 7(2), 95 - 102. 10.14744/ejmo.2023.42324 |
Chicago | Celik Selcen Epigenetic Code for Cell Fate During Development and Disease in Human. Eurasian Journal of Medicine and Oncology 7, no.2 (2023): 95 - 102. 10.14744/ejmo.2023.42324 |
MLA | Celik Selcen Epigenetic Code for Cell Fate During Development and Disease in Human. Eurasian Journal of Medicine and Oncology, vol.7, no.2, 2023, ss.95 - 102. 10.14744/ejmo.2023.42324 |
AMA | Celik S Epigenetic Code for Cell Fate During Development and Disease in Human. Eurasian Journal of Medicine and Oncology. 2023; 7(2): 95 - 102. 10.14744/ejmo.2023.42324 |
Vancouver | Celik S Epigenetic Code for Cell Fate During Development and Disease in Human. Eurasian Journal of Medicine and Oncology. 2023; 7(2): 95 - 102. 10.14744/ejmo.2023.42324 |
IEEE | Celik S "Epigenetic Code for Cell Fate During Development and Disease in Human." Eurasian Journal of Medicine and Oncology, 7, ss.95 - 102, 2023. 10.14744/ejmo.2023.42324 |
ISNAD | Celik, Selcen. "Epigenetic Code for Cell Fate During Development and Disease in Human". Eurasian Journal of Medicine and Oncology 7/2 (2023), 95-102. https://doi.org/10.14744/ejmo.2023.42324 |