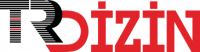
The Use of Plant Steroids in Viral Disease Treatments: Current Status and Future Perspectives
Yıl: 2023 Cilt: 82 Sayı: 1 Sayfa Aralığı: 86 - 94 Metin Dili: İngilizce DOI: 10.26650/EurJBiol.2023.1130357 İndeks Tarihi: 21-07-2023
The Use of Plant Steroids in Viral Disease Treatments: Current Status and Future Perspectives
Öz: Plants have been used for the prevention and treatment of diseases since the early days of humankind and constitute the natural sources of today’s modern medicine. Approximately one-quarter of approved drugs are derived from plants. Plant steroids are a group of biologically active secondary metabolites with a 5𝛼 and 5𝛽 gonane carbon skeleton. There is immense chemical diversity in plant steroids due to the side chains, oxidation status of the carbons in the tetracyclic core, and methyl groups. Plant steroids are classified into several groups based on their biological functions and structures, also on their mechanism of biosynthesis. All subtypes have been investigated for their anti-cancer, immunomodulatory, anti-inflammatory, and anti-viral properties. The novel coronavirus disease (COVID-19) is caused by severe acute respiratory syndrome coronavirus (SARS-CoV-2), which carries an RNA genome. An intense effort has been made in terms of effective treatment strategies and vaccine development since it was declared a pandemic. Nucleoside analogs such as favipiravir and remdesivir are used to block RNA-dependent RNA polymerase enzymes. Other strategies including neuraminidase inhibitors, chloroquine, and hydroxychloroquine as immunomodulatory agents, stem cell and cytokine based therapies are being conducted. One part of the therapies against SARS-CoV-2 is focused on the spike (S) protein of the virus that binds to the host receptor, angiotensin-converting enzyme 2 (ACE2). It has been suggested that SARS-CoV-2 S protein has a free fatty acid-binding pocket, and according to molecular simulations, steroids are ligands that bind to this pocket. Therefore, this review summarizes the plant steroid biological actions as well as their anti-viral potential against SARS-CoV-2 infection.
Anahtar Kelime: Belge Türü: Makale Makale Türü: Derleme Erişim Türü: Erişime Açık
- 1. Trautwein EA, McKay S. The role of specific components of a plant-based diet in management of dyslipidemia and the impact on cardiovascular risk. Nutrients. 2020; 12(9), 71-91.
- 2. Kreis W, Müller-Uri F. Biochemistry of sterols, cardiac glyco- sides, brassinosteroids, phytoecdysteroids and steroid saponins. Annu Rev Plant Biol. 2010;304–363.
- 3. Beg S, Hasan H, Hussain MS, et al. Systematic review of herbals as potential anti-inflammatory agents: Recent advances, cur- rent clinical status and future perspectives. Pharmacogn Rev. 2011;5(10):120-137.
- 4. Gunaherath GMK, Gunatilaka AAL. Plant steroids: occurrence, biological significance and their analysis. Encyc of Anal Chem. 2014;1–26.
- 5. Li Y, Li J, Zhou K, et al. A review on phytochemistry and phar- macology of cortex periplocae. Molecules. 2016;21(12):2702- 2718.
- 6. Bhandari J, Muhammad B, Thapa P, et al. Study of phyto- chemical, anti-microbial, anti-oxidant, and anti-cancer prop- erties of Allium wallichii. BMC Complement Altern Med. 2017;17(1):102.
- 7. Moosavi B, Liu S, Wang N, et al. The anti-fungal 𝛽-sitosterol tar- gets the yeast oxysterol-binding protein Osh4. Pest Management Science, 2019; 76(2):4–11.
- 8. Yang L, He J. Traditional uses, phytochemistry, pharmacology and toxicological aspects of the genus Hosta (Liliaceae): A com- prehensive review. J Ethnopharmacol. 2021; (265):113-123.
- 9. Grove MD, Spencer GF, Rohwedder WK, et al. Brassinolide, a plant growth-promoting steroid isolated from Brassica napus pollen. Nature. 1979;281(5728):216–217.
- 10. Yokota T, Takahashi N. Chemistry, physiology and agricultural application of brassinolide and related steroids. Proceed in Life Sci. 1986;129–138.
- 11. Kour J, Kohli SK, Khanna K, et al. Brassinosteroid sig- naling, crosstalk and, physiological functions in plants un- der heavy metal stress. Front Plant Sci. 2021;24(12):608061. doi:10.3389/fpls.2021.608061.
- 12. Khamsuk O, Sonjaroon W, Suwanwong S, et al. Effects of 24- epibrassinolide and the synthetic brassinosteroid mimic on chili pepper under drought. Acta Physiol. Plant. 2018;(40):106-118.
- 13. Sondhi N, Bhardwaj R, Kaur S, et al. Isolation of 24- epibrassinolide from leaves of Aegle marmelos and evaluation of its antigenotoxicity employing Allium cepa chromosomal aber- ration assay. Plant Growth Reg. 2007;54(3):217–224.
- 14. Carange J, Longpre F, Daoust B, et al. 24-epibrassinolide, a phytosterol from the brassinosteroid family, protects dopamin- ergic cells against MPP-induced oxidative stress and apoptosis. J Toxicol.2011:392859.
- 15. Clouse SD, Sasse JM. Brassinosteroids: Essential regulators of plant growth and development. Ann Rev Plant Physiol Plant Mol Biol. 1998;(49): 427-451.
- 16. Singh I, Shono M. Physiological and molecular effects of 24- epibrassinolide, a brassinosteroid on thermotolerance of tomato. Plant Grow Reg. 2005; 47(2–3): 111-119.
- 17. Coskun D, Obakan P, Arisan ED, et al. Epibrassinolide al- ters PI3K/MAPK signaling axis via activating Foxo3a-induced mitochondria-mediated apoptosis in colon cancer cells. Exp Cell Res. 2015; 15;338(1):10-21.
- 18. Ramirez JA, Michelini FM, Galagovsky LR, Berra A, Alche LE. Antiangiogenic brassinosteroid compounds. U.S. Patent 2013088400, 17 November 2015
- 19. Zhabinskii VN, Khripach NB, Khripach VA. Steroid plant hor- mones: effects outside plant kingdom. Steroids. 2015;(97):87- 97.
- 20. Kaur Kohli S, Bhardwaj A, Bhardwaj V, Sharma A, Kalia N, Landi M, et al. Therapeutic potential of brassinosteroids in biomedical and clinical research. Biomolecules. 2020;10(4):572.
- 21. Puschett JB, Agunanne E, Uddin MN. Emerging role of the bu- fadienolides in cardiovascular and kidney diseases. Am J Kidney Dis. 2010;56(2):359-370.
- 22. Tang HJ, Ruan LJ, Tian HY, et al. Novel stereoselective bufadienolides reveal new insights into the requirements for Na(+), K(+)-ATPase inhibition by cardiotonic steroids. Sci Rep. 2016;6:29155.
- 23. Gao H, Popescu R, Kopp B, Wang Z. Bufadienolides and their antitumor activity. Nat Prod Rep. 2011;28(5):953-969.
- 24. Li H, Cao X, Chen X, et al. Bufadienolides induce apopto- sis and autophagy by inhibiting the AKT signaling pathway in melanoma A-375 cells. Mol Med Rep. 2019;20(3):2347-2354.
- 25. Kaushik U, Aeri V, Mir SR. Cucurbitacins-An insight into medicinal leads from nature. Pharmacogn Rev. 2015;9(17):12- 18.
- 26. Oi T, Asanuma K, Matsumine A, et al. STAT3 inhibitor, cucur- bitacin I, is a novel therapeutic agent for osteosarcoma. Int J Oncol. 2016; 49(6):2275-2284.
- 27. Chen X, Bao J, Guo J, et al. Biological activities and potential molecular targets of cucurbitacins: a focus on cancer. Anticancer Drugs. 2012; 23(8):777-787.
- 28. Dinan L. Phytoecdysteroids: biological aspects. Phytochemistry. 2001;57(3):325-339.
- 29. Chadin I, Volodin V, Whiting P, Shirshova T, Kolegova N, Di- nan L. Ecdysteroid content and distribution in plants of genus Potamogeton, Biochem Syst and Ecol. 2003; 31(4): 407-415.
- 30. Lafont R, Dinan L. Practical uses for ecdysteroids in mammals including humans: an update . J Insect Sci. 2003;3:7.
- 31. Festucci-Buselli RA, Contim LA, Barbosa LCA, Stuart J, Otoni WC. Biosynthesis and potential functions of the ecdysteroid 20- hydroxyecdysone-a review. Botany. 2008;86(9): 978-987.
- 32. Shakhmurova GA, Mamadalieva NZ, Zhanibekov AA, Khush- baktova ZA, Syrov VN. Effect of total ecdysteroid preparation from Silene viridiflora on the immune state of experimental ani- mals under normal and secondary immunodeficiency conditions. Pharm Chem J. 2012;46 (4), 222–224.
- 33. Ishola, IO, Ochieng, CO, Olayemi SO, Jimoh MO, Lawal SM. Potential of novel phytoecdysteroids isolated from Vitex doniana in the treatment depression: Involvement of monoaminergic sys- tems. Pharmacol Biochem Behav. 2014;127, 90–100.
- 34. Kregiel D, Berlowska J, Witonska I, et al. Saponin-based, biological-active surfactants from plants. In application and characterization of surfactants. Edited by Reza Najjar InTech. doi: 10.5772/65591, 2017
- 35. Marrelli M, Conforti F, Araniti F, et al. Effects of saponins on lipid metabolism: a review of potential health benefits in the treatment of obesity. Molecules. 2016;21(10),1404.
- 36. Du JR, Long FY, Chen C. Research progress on natural triter- penoid saponins in the chemoprevention and chemotherapy of cancer. Enzymes. 2014;(36):95-130.
- 37. Sarkar FH, Li Y, Wang Z, et al. Cellular signaling perturbation by natural products. Cell Signal. 2009;21(11):1541-1547.
- 38. Haridas V, Higuchi M, Jayatilake GS, et al. Avicins: triter- penoid saponins from Acacia victoriae (Bentham) induce apop- tosis by mitochondrial perturbation. Proc Natl Acad Sci USA. 2001;98(10):5821-5826.
- 39. Patlolla JM, Raju J, Swamy MV, et al. Beta-escin inhibits colonic aberrant crypt foci formation in rats and regulates the cell cycle growth by inducing p21(waf1/cip1) in colon cancer cells. Mol Cancer Ther. 2006;5(6):1459-1466.
- 40. Fukumura M, Ando H, Hirai Y, et al. Achyranthoside H methyl ester, a novel oleanolic acid saponin derivative from Achyranthes fauriei roots, induces apoptosis in human breast cancer MCF-7 and MDA-MB-453 cells via a caspase activation pathway. J Nat Med. 2009;63(2):181-188.
- 41. Ooh KF, Ong HC, Wong FC, et al. High performance liquid chromatography profiling of health-promoting phytochemicals and evaluation of antioxidant, anti-lipoxygenase, iron chelat- ing and anti- glucosidase activities of wetland macrophytes. Pharmacogn Mag. 2014;10(3):443-455.
- 42. Dey P, Kundu A, Chakraborty HJ, et al. Therapeutic value of steroidal alkaloids in cancer: Current trends and future perspec- tives. Int J Cancer. 2019;145(7):1731-1744.
- 43. Dirsch VM, Müller IM, Eichhorst ST, et al. Cephalostatin 1 selectively triggers the release of Smac/DIABLO and subsequent apoptosis that is characterized by an increased density of the mitochondrial matrix. Cancer Res. 2003;63(24):8869-8876.
- 44. Moser BR. Review of cytotoxic cephalostatins and ritterazines: isolation and synthesis. J Nat Prod. 2008;71(3):487-491.
- 45. Lacour TG, Guo C, Bhandaru S, et al. Interphylal Product Splic- ing: The First Total Syntheses of Cephalostatin 1, the North Hemisphere of Ritterazine G, and the Highly Active Hybrid Analogue, Ritterostatin GN1N1. J Am Chem Soc. 1998; 120(4): 692–707.
- 46. Torres MC, das Chagas L Pinto F, et al. Antiophidic solanidane steroidal alkaloids from Solanum campaniforme. J Nat Prod. 2011;74(10):2168-2173.
- 47. Wang K, Sasaki T, Li W, et al. Two novel steroidal alkaloid glycosides from the seeds of Lycium barbarum. Chem Biodivers. 2011;8(12):2277-2284.
- 48. Jan NU, Ahmad B, Ali S, et al. Steroidal alkaloids as an emerg- ing therapeutic alternative for investigation of their immunosup- pressive and hepatoprotective potential. Front Pharmacol. 2017; (8):114.
- 49. Antony ML, Lee J, Hahm ER, et al. Growth arrest by the an- titumor steroidal lactone withaferin A in human breast cancer cells is associated with down-regulation and covalent binding at cysteine 303 of 𝛽-tubulin. J Biol Chem. 2014;289(3):1852-1865.
- 50. Zhang H, Samadi AK, Gallagher RJ, et al. Cytotoxic with- anolide constituents of Physalis longifolia. J Nat Prod. 2011;74(12):2532-2544.
- 51. Zhang H, Bazzill J, Gallagher RJ, et al. Antiproliferative with- anolides from Datura wrightii. J Nat Prod. 2013;76(3):445-449.
- 52. Grogan PT, Sleder KD, Samadi AK, et al. Cytotoxicity of withaferin A in glioblastomas involves induction of an ox- idative stress-mediated heat shock response while altering Akt/mTOR and MAPK signaling pathways. Invest New Drugs. 2013;31(3):545-557.
- 53. Srigley CT, Haile EA. Quantification of plant sterols/stanols in foods and dietary supplements containing added phytosterols. J Food Comp Anal. 2015;(40):163–176.
- 54. Miras-Moreno B, Sabater-Jara AB, Pedreno MA, et al. Bioactiv- ity of phytosterols and their production in plant in vitro cultures. J Agric Food Chem. 2016; 64(38):7049-7058.
- 55. Salehi-Sahlabadi A, Varkaneh HK, Shahdadian F, et al. Effects of Phytosterols supplementation on blood glucose, glycosylated hemoglobin (HbA1c) and insulin levels in humans: a system- atic review and meta-analysis of randomized controlled trials. J Diabetes Metab. Disord. 2020;19(1):625-632.
- 56. Moreau RA, Nystrom L, Whitaker BD, et al. Phytos- terols and their derivatives: Structural diversity, distribution, metabolism, analysis, and health-promoting uses. Prog Lipid Res. 2018;(70):35-61
- 57. Hannan MA, Sohag AAM, Dash R, et al. Phytosterols of marine algae: Insights into the potential health benefits and molecular pharmacology. Phytomedicine. 2020;(69):153201.
- 58. Vezza T, Canet F, de Maranon AM, et al. Phytosterols: Nutri- tional health players in the management of obesity and its related disorders. Antioxidants (Basel). 2020;9(12):1266.
- 59. Liao PC, Lai MH, Hsu KP, et al. Identification of 𝛽-Sitosterol as in vitro anti-inflammatory constituent in Moringa oleifera. J Agric Food Chem. 2018;66(41):10748-10759.
- 60. Turnbaugh PJ, Backhed F, Fulton L, et al. Diet-induced obesity is linked to marked but reversible alterations in the mouse distal gut microbiome. Cell Host Microbe. 2008;3(4):213-223.
- 61. Martínez I, Perdicaro DJ, Brown AW, et al. Diet-induced alter- ations of host cholesterol metabolism are likely to affect the gut microbiota composition in hamsters. Appl Environ Microbiol. 2013;79(2):516-524.
- 62. Castilla V, Ramirez J, Coto CE. Plant and animal steroids a new hope to search for antiviral agents. Curr Med Chem. 2010;17(18):1858-1873.
- 63. Denaro M, Smeriglio A, Barreca D, et al. Antiviral activity of plants and their isolated bioactive compounds: An update. Phytother Res. 2020;34(4):742-768.
- 64. Alkhatib A, Tsang C, Tiss A, et al. Functional foods and lifestyle approaches for diabetes prevention and management. Nutrients. 2017;9(12):1310.
- 65. Parvez MK, Alam P, Arbab AH, et al. Analysis of antioxidative and antiviral biomarkers 𝛽-amyrin, 𝛽-sitosterol, lupeol, ursolic acid in Guiera senegalensis leaves extract by validated HPTLC methods. Saudi Pharm J. 2018;26(5):685-693.
- 66. Zhou BX, Li J, Liang XL, et al. 𝛽-sitosterol ameliorates influenza A virus-induced proinflammatory response and acute lung in- jury in mice by disrupting the cross-talk between RIG-I and IFN/STAT signaling. Acta Pharmacol Sin. 2020;41(9):1178- 1196.
- 67. Yuan M, Jiang Z, Bi G, et al. Pattern-recognition recep- tors are required for NLR-mediated plant immunity. Nature. 2021;592(7852):105-109.
- 68. Calil IP, Fontes EPB. Plant immunity against viruses: antiviral immune receptors in focus. Ann Bot. 2017;119(5):711-723.
- 69. Wachsman MB, Lopez EM, Ramirez JA, et al. Antiviral effect of brassinosteroids against herpes virus and arenaviruses. Antivir Chem Chemother. 2000;11(1):71-77.
- 70. Nakashita H, Yasuda M, Nitta T, et al. Brassinosteroid functions in a broad range of disease resistance in tobacco and rice. Plant J. 2003; 33(5):887-898.
- 71. Yang H, Gou X, He K, et al. BAK1 and BKK1 in Arabidopsis thaliana confer reduced susceptibility to turnip crinkle virus. Eur J Plant Pathol. 2010; 127(1),149–156.
- 72. Zhang DW, Deng XG, Fu FQ, et al. Induction of plant virus de- fense response by brassinosteroids and brassinosteroid signaling in Arabidopsis thaliana. Planta. 2015; 241(4):875-885.
- 73. Shamsabadipour S, Ghanadian M, Saeedi H, et al. Triterpenes and steroids from Euphorbia denticulata Lam. with anti-herpes symplex virus activity. Iran J Pharm Res. 2013;12(4):759-767.
- 74. Supratman U, Fujita T, Akiyama K, et al. Insecticidal compounds from Kalanchoe daigremontiana x tubiflora. Phytochemistry. 2001;58(2):311-314.
- 75. Munakarmi S, Chand L, Shin HB, et al. Anticancer effects of Poncirus fructus on hepatocellular carcinoma through reg- ulation of apoptosis, migration, and invasion. Oncol Rep. 2020;44(6):2537-2546
- 76. Liu Y, Yang H, Guo Q, et al. Cucurbitacin E Inhibits Huh7 Hepatoma Carcinoma Cell Proliferation and Metastasis via Suppressing MAPKs and JAK/STAT3 Pathways. Molecules. 2020;25(3):560.
- 77. Troost B, Mulder LM, Diosa-Toro M, et al. Tomatidine, a natural steroidal alkaloid shows antiviral activity towards chikungunya virus in vitro. Sci Rep. 2020;10(1):6364.
- 78. Wang P, Bai J, Liu X, et al. Tomatidine inhibits porcine epidemic diarrhea virus replication by targeting 3CL protease. Vet Res. 2020;51(1):136.
- 79. Brian DA, Baric RS. Coronavirus genome structure and replica- tion. Curr Top Microbiol Immunol. 2005;(287):1-30.
- 80. Fehr AR, Perlman S. Coronaviruses: an overview of their repli- cation and pathogenesis. Methods Mol Biol. 2015;(1282):1-23.
- 81. Mahmood N, Nasir SB, Hefferon K. Plant-Based Drugs and Vaccines for COVID-19. Vaccines (Basel). 2020;9(1):15.
- 82. Dent SD, Xia D, Wastling JM, et al. The proteome of the infectious bronchitis virus Beau-R virion. J Gen Virol. 2015;96(12):3499-3506.
- 83. Wu F, Zhao S, Yu B, et al. A new coronavirus associated with hu- man respiratory disease in China. Nature. 2020;579(7798):265- 269.
- 84. Tang X, Wu C, Li X, et al. On the origin and continuing evolution of SARS-CoV-2. Natl Sci Rev.2020;7(6):1012-1023.
- 85. Corman VM, Muth D, Niemeyer D, et al. Hosts and Sources of Endemic Human Coronaviruses. Adv Virus Res. 2018;(100):163-188.
- 86. van Paassen J, Vos JS, Hoekstra EM, et al. Corticosteroid use in COVID-19 patients: a systematic review and meta-analysis on clinical outcomes. Crit Care. 2020;24(1):696.
- 87. Fischer R, Buyel JF. Molecular farming-The slope of enlighten- ment. Biotechnol. Adv. 2020; (40):107519.
- 88. Capell T, Twyman RM, Armario-Najera V, et al. Potential ap- plications of plant biotechnology against SARS-CoV-2. Trends Plant Sci. 2020 25(7):635-643.
- 89. Shang J, Ye G, Shi K, et al. Structural basis of receptor recogni- tion by SARS-CoV-2. Nature. 2020; 581(7807):221-224.
- 90. Bhuiyan FR, Howlader S, Raihan T, et al. Plants metabolites: Possibility of natural therapeutics against the COVID-19 pan- demic. Front Med (Lausanne). 2020;(7):444.
- 91. Fernandez-Oliva A, Ortega-Gonzalez P, Risco C. Targeting host lipid flows: Exploring new antiviral and antibiotic strategies. Cell Microbiol. 2019;21(3):e12996.
- 92. Baglivo M, Baronio M, Natalini G, et al. Natural small molecules as inhibitors of coronavirus lipid-dependent attachment to host cells: a possible strategy for reducing SARS-COV-2 infectivity? Acta Biomed. 2020;91(1):161-164.
- 93. Li SY, Chen C, Zhang HQ, et al. Identification of natural com- pounds with antiviral activities against SARS-associated coron- avirus. Antiviral Res. 2005;67(1):18-23.
- 94. Cheng PW, Ng LT, Chiang LC, et al. Antiviral effects of saikos- aponins on human coronavirus 229E in vitro. Clin Exp Pharma- col Physiol. 2006;33(7):612-616.
- 95. Yang CW, Lee YZ, Hsu HY, et al. Inhibition of SARS-CoV-2 by highly potent broad-spectrum anti-coronaviral tylophorine- based derivatives. Front Pharmacol. 2020;(11):606097.
- 96. Puttaswamy H, Gowtham HG, Ojha MD, et al. In silico stud- ies evidenced the role of structurally diverse plant secondary metabolites in reducing SARS-CoV-2 pathogenesis. Sci Rep. 2020;10(1):20584.
- 97. Shoemark DK, Colenso CK, Toelzer C, et al. Molecular simu- lations suggest vitamins, retinoids and steroids as ligands of the free fatty acid pocket of the SARS-CoV-2 spike protein. Angew Chem Int Ed Engl. 2021; 60(13):7098-8010.
- 98. Alka H, Akhilesh A, Archana S, et al. Cucurbitacin: As a candi- date against cytokine storm in severe COVID-19 infection. Int J Res Pharm Sci. 2020;11(1): 928–930.
- 99. Conti P, Ronconi G, Caraffa A, et al. Induction of pro- inflam- matory cytokines (IL-1 and IL-6) and lung inflammation by Coronavirus-19 (COVI-19 or SARS-CoV-2): anti-inflammatory strategies. J Biol Regul Homeost Agents. 2020;34(2):327-331.
- 100. Qiao J, Xu LH, He J, et al. Cucurbitacin E exhibits anti- inflammatory effect in RAW 264.7 cells via suppression of NF- 𝜅B nuclear translocation. Inflamm Res. 2013;62(5):461-469.
- 101. Escandell JM, Recio MC, Manez S, et al. Cucurbitacin R reduces the inflammation and bone damage associated with adjuvant arthritis in lewis rats by suppression of tumor necrosis factor- alpha in T lymphocytes and macrophages. J Pharmacol Exp Ther. 2007;320(2):581-590.
APA | OBAKAN YERLİKAYA P, ARISAN E, MEHDIZADEHTAPEH L, ONGANER P, GURKAN A (2023). The Use of Plant Steroids in Viral Disease Treatments: Current Status and Future Perspectives. , 86 - 94. 10.26650/EurJBiol.2023.1130357 |
Chicago | OBAKAN YERLİKAYA Pınar,ARISAN Elif Damla,MEHDIZADEHTAPEH Leila,ONGANER Pınar UYSAL,GURKAN Ajda COKER The Use of Plant Steroids in Viral Disease Treatments: Current Status and Future Perspectives. (2023): 86 - 94. 10.26650/EurJBiol.2023.1130357 |
MLA | OBAKAN YERLİKAYA Pınar,ARISAN Elif Damla,MEHDIZADEHTAPEH Leila,ONGANER Pınar UYSAL,GURKAN Ajda COKER The Use of Plant Steroids in Viral Disease Treatments: Current Status and Future Perspectives. , 2023, ss.86 - 94. 10.26650/EurJBiol.2023.1130357 |
AMA | OBAKAN YERLİKAYA P,ARISAN E,MEHDIZADEHTAPEH L,ONGANER P,GURKAN A The Use of Plant Steroids in Viral Disease Treatments: Current Status and Future Perspectives. . 2023; 86 - 94. 10.26650/EurJBiol.2023.1130357 |
Vancouver | OBAKAN YERLİKAYA P,ARISAN E,MEHDIZADEHTAPEH L,ONGANER P,GURKAN A The Use of Plant Steroids in Viral Disease Treatments: Current Status and Future Perspectives. . 2023; 86 - 94. 10.26650/EurJBiol.2023.1130357 |
IEEE | OBAKAN YERLİKAYA P,ARISAN E,MEHDIZADEHTAPEH L,ONGANER P,GURKAN A "The Use of Plant Steroids in Viral Disease Treatments: Current Status and Future Perspectives." , ss.86 - 94, 2023. 10.26650/EurJBiol.2023.1130357 |
ISNAD | OBAKAN YERLİKAYA, Pınar vd. "The Use of Plant Steroids in Viral Disease Treatments: Current Status and Future Perspectives". (2023), 86-94. https://doi.org/10.26650/EurJBiol.2023.1130357 |
APA | OBAKAN YERLİKAYA P, ARISAN E, MEHDIZADEHTAPEH L, ONGANER P, GURKAN A (2023). The Use of Plant Steroids in Viral Disease Treatments: Current Status and Future Perspectives. European Journal of Biology, 82(1), 86 - 94. 10.26650/EurJBiol.2023.1130357 |
Chicago | OBAKAN YERLİKAYA Pınar,ARISAN Elif Damla,MEHDIZADEHTAPEH Leila,ONGANER Pınar UYSAL,GURKAN Ajda COKER The Use of Plant Steroids in Viral Disease Treatments: Current Status and Future Perspectives. European Journal of Biology 82, no.1 (2023): 86 - 94. 10.26650/EurJBiol.2023.1130357 |
MLA | OBAKAN YERLİKAYA Pınar,ARISAN Elif Damla,MEHDIZADEHTAPEH Leila,ONGANER Pınar UYSAL,GURKAN Ajda COKER The Use of Plant Steroids in Viral Disease Treatments: Current Status and Future Perspectives. European Journal of Biology, vol.82, no.1, 2023, ss.86 - 94. 10.26650/EurJBiol.2023.1130357 |
AMA | OBAKAN YERLİKAYA P,ARISAN E,MEHDIZADEHTAPEH L,ONGANER P,GURKAN A The Use of Plant Steroids in Viral Disease Treatments: Current Status and Future Perspectives. European Journal of Biology. 2023; 82(1): 86 - 94. 10.26650/EurJBiol.2023.1130357 |
Vancouver | OBAKAN YERLİKAYA P,ARISAN E,MEHDIZADEHTAPEH L,ONGANER P,GURKAN A The Use of Plant Steroids in Viral Disease Treatments: Current Status and Future Perspectives. European Journal of Biology. 2023; 82(1): 86 - 94. 10.26650/EurJBiol.2023.1130357 |
IEEE | OBAKAN YERLİKAYA P,ARISAN E,MEHDIZADEHTAPEH L,ONGANER P,GURKAN A "The Use of Plant Steroids in Viral Disease Treatments: Current Status and Future Perspectives." European Journal of Biology, 82, ss.86 - 94, 2023. 10.26650/EurJBiol.2023.1130357 |
ISNAD | OBAKAN YERLİKAYA, Pınar vd. "The Use of Plant Steroids in Viral Disease Treatments: Current Status and Future Perspectives". European Journal of Biology 82/1 (2023), 86-94. https://doi.org/10.26650/EurJBiol.2023.1130357 |