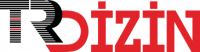
Potential improvement of clinker sand in the mechanical high temperature and transport properties with GGBS-based prepacked geopolymer composite
Yıl: 2023 Cilt: 9 Sayı: 3 Sayfa Aralığı: 92 - 106 Metin Dili: İngilizce DOI: 10.20528/cjsmec.2023.03.002 İndeks Tarihi: 15-09-2023
Potential improvement of clinker sand in the mechanical high temperature and transport properties with GGBS-based prepacked geopolymer composite
Öz: In this study, a new generation prepacked geopolymer composite (PGC) material that can meet different needs was obtained by combining geopolymer concrete (GPC) and prepacked aggregate concrete (PAC) technology. In the production of PGC, 5-8 mm quartz aggregates were placed in molds and, geopolymer mortar was injected be-tween these aggregates. Aluminosilicate based blast furnace slag (GBFS) was used as binding in geopolymer mortars; sodium silicate (Na2SiO3) and sodium hydroxide (NaOH) was used as alkali activator. In addition, clinker aggregate in different propor-tions was used as fine aggregate (0–4 mm) in the production of mortar. Within the scope of the study, the physical, mechanical, permeability and high temperature re-sistance properties of PGC were investigated. The produced samples were cured at 30 and 60 °C for 6 and 8 hours. Hardened unit weights of PGC vary between 2342 and 2539 kg/m3, and sorptivity values vary between 0 and 0.04 kg/m2.min0.5. While the increase in curing temperature and curing time increases the hardened unit weight values, it decreased permeability values. While the increase of clinker aggregate in the mortar phase does not change the hardened unit weight, it significantly reduced per-meability. When the PGC samples are cured at 30 °C, the compressive strengths are 20.38–39.03 MPa; when curing at 60 °C, the compressive strengths are 35.21–57.04 MPa. Flexural strengths, 2.35–6.96 MPa with 30 °C cure, achieved 4.27–9.93 MPa results with 60 °C curing. Increasing the curing time and curing temperature signifi-cantly increased the compressive and flexural strengths. The increase in the amount of clinker aggregate added to the mortar phase decreased the strength values. While the compressive strength values of PGC mixtures do not fall below 15 MPa after being exposed to 300 °C high temperature; after the application of 600 °C high temperature, it lost up to 70% of its strength. Increasing the curing time and curing temperature, increased the high temperature resistance. The increase in the amount of clinker ag-gregate in the mixtures, decreased the strength loss rate.
Anahtar Kelime: Belge Türü: Makale Makale Türü: Araştırma Makalesi Erişim Türü: Erişime Açık
- Ahmed HU, Mohammed AS, Qaidi SM, Faraj RH, Hamah Sor N, Mohammed A (2022). Compressive strength of geopolymer concrete composites: a systematic comprehensive review, analysis and modeling. European Journal of Environmental and Civil Engineering, 1-46.
- Aı̈tcin PC (2000). Cements of yesterday and today: concrete of tomorrow. Cement and Concrete Research, 30(9), 1349-1359.
- Albitar M., Ali MM, Visintin P, Drechsler M (2017). Durability evaluation of geopolymer and conventional concretes. Construction and Building Materials, 136, 374-385.
- Alcan HG, Bayrak B, Öz A, Kavaz E, Kaplan G, Çelebi O, Aydın AC (2023). A comprehensive characterization on geopolymer concretes with low content slag and quartz aggregates: the shielding features. Radiation Effects and Defects in Solids, 1-30.
- Aldanmaz BA (2020). Karma Lifli betonların tek eksenli çekme altında çatlak yapısının incelenmesi. Teknik Dergi, 31(1), 9773-9787.
- Amran M, Debbarma S, Ozbakkaloglu T (2021). Fly ash-based eco-friendly geopolymer concrete: A critical review of the long-term durability properties. Construction and Building Materials, 270, 121857.
- Aredes F, Campos T, Machado J, Sakane K, Thim G, Brunelli D (2015). Effect of cure temperature on the formation of metakaolinite-based geopolymer. Ceramics International, 41(6), 7302-7311.
- Arioz O (2009). Retained properties of concrete exposed to high temperatures: Size effect. Fire and Materials: An International Journal, 33(5), 211-222.
- ASTM C348 (2008). Standard test method for flexural strength of hydraulic-cement mortars. ASTM C348-02, Annu book ASTM Stand 04.01.
- ASTM C349 (2008). Standard test method for compressive strength of hydraulic cement mortar (using portions of prisms broken in flexure), West Conshohocken (PA): ASTM International.
- ASTM C642 (2006). Standard test method for density, absorption, and voids in hardened concrete. American Society for Testing of Materials, Annual Book of ASTM Standards, vol. 04.02, Philadelphia.
- ASTM C989 (2006). Standard specification for ground granulated blast furnace slag for use in concrete and mortars. vol. 4.02.
- Aydin AC, Arslan A, Gül R (2007). Mesoscale simulation of cement based materials’ time-dependent behavior. Computational Materials Science, 41(1), 20-26.
- Barcelo L, Kline J, Walenta G, Gartner E (2014). Cement and carbon emissions. Materials and Structures, 47(6), 1055-1065.
- Behnood A. Ghandehari M, (2009). Comparison of compressive and splitting tensile strength of high-strength concrete with and without polypropylene fibers heated to high temperatures. Fire Safety Journal, 44(8), 1015-1022.
- Benhelal E, Zahedi G, Shamsaei E, Bahadori A (2013). Global strategies and potentials to curb CO2 emissions in cement industry. Journal of Cleaner Production, 51, 142-161.
- Berger R (1974). Properties of concrete with cement clinker aggregate. Cement and Concrete Research, 4(1), 99-112.
- Beshr H, Almusallam A, Maslehuddin M, (2003). Effect of coarse aggregate quality on the mechanical properties of high strength concrete. Construction and Building Materials, 17(2), 97-103.
- Chan Y, Peng G, Anson M (1999). Residual strength and pore structure of high-strength concrete and normal strength concrete after exposure to high temperatures. Cement and Concrete Composites, 21(1), 23-27.
- Cheng Y, Liu S, Zhu B, Liu R, Wang Y (2019). Preparation of preplaced aggregate concrete and experimental study on its strength. Construction and Building Materials, 229, 116847.
- Das KK, Lam ES, Tang HH (2021). Partial replacement of cement by ground granulated blast furnace slag and silica fume in two stage concrete (preplaced aggregate concrete). Structural Concrete, 22, E466-E473.
- De Castro S, de Brito J (2013). Evaluation of the durability of concrete made with crushed glass aggregates. Journal of Cleaner Production, 41, 7-14.
- Domingo-Cabo A, Lázaro C, López-Gayarre F, Serrano-López M, Serna P, Castaño-Tabares JO (2009). Creep and shrinkage of recycled aggregate concrete. Construction and Building Materials, 23(7), 2545-2553.
- Durak U (2022). Effect of short-term elevated temperature curing on strength properties and microstructure of fresh fly ash geopolymer mortar. Arabian Journal of Geosciences, 15(9), 810.
- EN 1015-18 (2002). Methods of Test for Mortar for Masonry—Part 18: Determination of Water Absorption Coefficient Due to Capillary Action of Hardened Mortar.
- Golewski GL (2021). Green concrete based on quaternary binders with significant reduced of CO2 emissions. Energies, 14(15), 4558.
- Gültekin A, Hosseinnezhad H, Ramyar K (2022). Agrega türünün geopolimer betonun yüksek sıcaklık direncine etkisi. Politeknik Dergisi, 1-1.
- Güzelküçük S, Demir İ (2019). Perlit Esaslı Geopolimer Kompozitlere Kür Süresi Ve Sıcaklığın Etkisi. International Journal of Engineering Research and Development, 11(2), 730-737.
- Hager I, Sitarz M, Mróz K (2021). Fly-ash based geopolymer mortar for high-temperature application–Effect of slag addition. Journal of Cleaner Production, 316, 128168.
- Hassan A, Arif M, Shariq M (2019). Effect of curing condition on the mechanical properties of fly ash-based geopolymer concrete. SN Applied Sciences, 1, 1-9.
- Huo J, Zheng Q, Chen B, Xiao Y (2009). Tests on impact behaviour of micro-concrete-filled steel tubes at elevated temperatures up to 400 C. Materials and Structures, 42, 1325-1334.
- Jindal BB, Alomayri T, Hasan A, Kaze CR (2022). Geopolymer concrete with metakaolin for sustainability: a comprehensive review on raw material’s properties, synthesis, performance, and potential application. Environmental Science and Pollution Research, 1-26.
- Kaplan G, Shi J, Öz A, Bayrak B, M. Dheyaaldin MH, Aydın AC (2023). Preparation and characterization of a novel prepacked aggregate geopolymer: A feasibility study. Powder Technology, 421, 118423.
- Khalid HR, Lee N, Choudhry I, Wang Z, Lee HK (2019). Evolution of zeolite crystals in geopolymer-supported zeolites: Effects of composition of starting materials. Materials Letters, 239, 33-36.
- Khayat KH (1999). Workability, testing, and performance of self-consolidating concrete. Materials Journal, 96(3), 346-353.
- Krishna R, Mishra J, Zribi M, Adeniyi F, Saha S, Baklouti S, Shaikh FUA Gökçe H (2021). A review on developments of environmentally friendly geopolymer technology. Materialia, 20, 101212.
- Kürklü G (2016). The effect of high temperature on the design of blast furnace slag and coarse fly ash-based geopolymer mortar. Composites Part B: Engineering, 92, 9-18.
- Lv J, Zhou T, Li K (2020). Development and investigation of a new low-cement-consumption concrete—Preplaced aggregate concrete. Sustainability, 12(3), 1080.
- Mermerdaş K, Manguri S, Nassani DE, Oleiwi SM (2017). Effect of aggregate properties on the mechanical and absorption characteristics of geopolymer mortar. Engineering Science and Technology, An International Journal, 20(6), 1642-1652.
- Meyer CJC (2009). The greening of the concrete industry. Cement and Concrete Composites, 31(8), 601-605.
- Mo B, Zhu H, Cui X, He Y, Gong S (2014). Effect of curing temperature on geopolymerization of metakaolin-based geopolymers. Applied Clay Science, 99, 144-148.
- Mohammed AA, Ahmed HU, Mosavi A (2021). Survey of mechanical properties of geopolymer concrete: a comprehensive review and data analysis. Materials, 14(16), 4690.
- Noumowe A, (2005). Mechanical properties and microstructure of high strength concrete containing polypropylene fibres exposed to temperatures up to 200 C. Cement and Concrete Research, 35(11), 2192-2198.
- Noushini A, Castel A (2016). The effect of heat-curing on transport properties of low-calcium fly ash-based geopolymer concrete. Construction and Building Materials, 112, 464-477.
- Nuaklong P, Worawatnalunart P, Jongvivatsakul P, Tangaramvong S, Pothisiri T, Likitlersuang S (2021). Pre-and post-fire mechanical performances of high calcium fly ash geopolymer concrete containing granite waste. Journal of Building Engineering, 44, 103265.
- Öz A, Bayrak B, Kavaz E, Kaplan G, Çelebi O, Alcan HG, Aydın AC (2022). The radiation shielding and microstructure properties of quartzic and metakaolin based geopolymer concrete. Construction and Building Materials, 342, 127923.
- Prokopski G, Halbiniak J (2000). Interfacial transition zone in cementitious materials. Cement and Concrete Research, 30(4), 579-583.
- Raza A, Ouni MHE, Azab M, Ali K, Haider H, Rashedi A (2022). A scientometric review on mechanical and durability performance of geopolymer Paste: Effect of various raw materials. Construction and Building Materials, 345, 128297.
- Samal S, (2019). Effect of high temperature on the microstructural evolution of fiber reinforced geopolymer composite. Heliyon, 5(5), e01779.
- Schneider U, (1988). Concrete at high temperatures—a general review. Fire Safety Journal, 13(1), 55-68.
- Sevinç AH, Durgun MY (2020). Properties of high-calcium fly ash-based geopolymer concretes improved with high-silica sources. Construction and Building Materials, 261, 120014.
- Shafaghat J, Allahverdi A (2019). Enhancing concrete properties by using silica fume as reactive powder and portland cement-clinker as reactive aggregate. Journal of Materials in Civil Engineering, 31(11), 04019278.
- Shaikh FUA (2016). Mechanical and durability properties of fly ash geopolymer concrete containing recycled coarse aggregates. International Journal of Sustainable Built Environment, 5(2), 277-287.
- Suhendro B (2014). Toward green concrete for better sustainable environment. Procedia Engineering, 95: 305-320.
- Sukontasukkul P, Pomchiengpin W, Songpiriyakij S (2010). Post-crack (or post-peak) flexural response and toughness of fiber reinforced concrete after exposure to high temperature. Construction and Building Materials, 24(10), 1967-1974.
- Tayeh BA, Zeyad AM, IAgwa IS, Amin M (2021). Effect of elevated temperatures on mechanical properties of lightweight geopolymer concrete. Case Studies in Construction Materials, 15: e00673.
- Titi HH, Tabatabai H (2018). Effect of coarse aggregate type on chloride ion penetration in concrete. Construction and Building Materials, 162, 871-880.
- Topçu İB, Canbaz M (2008). Silis dumanının betonda mekanik çatlak oluşumlarına etkisi. Eskişehir Osmangazi Üniversitesi Mühendislik ve Mimarlık Fakültesi Dergisi, 21(2), 17-26.
- Van Deventer JS, Provis JL, Duxson P, Brice DG (2010). Chemical research and climate change as drivers in the commercial adoption of alkali activated materials. Waste and Biomass Valorization, 1, 145-155.
- Van Jaarsveld J, Van Deventer JS, Lukey G (2002). The effect of composition and temperature on the properties of fly ash-and kaolinite-based geopolymers. Chemical Engineering Journal, 89(1-3), 63-73.
- Venkatesan RP, Pazhani K (2016). Strength and durability properties of geopolymer concrete made with ground granulated blast furnace slag and black rice husk ash. KSCE Journal of Civil Engineering, 20, 2384-2391.
- Verma M, Dev N (2022). Effect of ground granulated blast furnace slag and fly ash ratio and the curing conditions on the mechanical properties of geopolymer concrete. Structural Concrete, 23(4), 2015-2029.
- Wakeley L, Roy D (1983). Experimental concretes for sealing radioactive-waste repositories in evaporite strata. Cement and Concrete Research, 13(1), 97-106.
- Wasim M, Ngo TD, Law D (2021). A state-of-the-art review on the durability of geopolymer concrete for sustainable structures and infrastructure. Construction and Building Materials, 291, 123381.
- Winnefeld F, Leemann A, German A, Lothenbach B (2022). CO2 storage in cement and concrete by mineral carbonation. Current Opinion in Green and Sustainable Chemistry, 100672.
- Worrell E, Price L, Martin N, Hendriks C, Meida LO (2001). Carbon dioxide emissions from the global cement industry. Annual Review of Energy and the Environment, 26(1), 303-329.
- Yoon J, Kim H, Shin SW, Sim SH (2020). Rheology-based determination of injectable grout fluidity for preplaced aggregate concrete using ultrasonic tomography. Construction and Building Materials, 260, 120447.
- Zhang C, Wang X, Hu Z, Wu Q, Zhu H, Lu J (2021). Long-term performance of silane coupling agent/metakaolin based geopolymer. Journal of Building Engineering, 36, 102091.
- Zhang G, Yang H, Ju C, Yang Y (2020). Novel selection of environment-friendly cementitious materials for winter construction: Alkali-activated slag/Portland cement. Journal of Cleaner Production, 258, 120592.
APA | ALCAN H, Aksu Alcan B, BAYRAK B, AYDIN A (2023). Potential improvement of clinker sand in the mechanical high temperature and transport properties with GGBS-based prepacked geopolymer composite. , 92 - 106. 10.20528/cjsmec.2023.03.002 |
Chicago | ALCAN HALUK GÖRKEM,Aksu Alcan Bilge,BAYRAK Barış,AYDIN Abdulkadir Cuneyt Potential improvement of clinker sand in the mechanical high temperature and transport properties with GGBS-based prepacked geopolymer composite. (2023): 92 - 106. 10.20528/cjsmec.2023.03.002 |
MLA | ALCAN HALUK GÖRKEM,Aksu Alcan Bilge,BAYRAK Barış,AYDIN Abdulkadir Cuneyt Potential improvement of clinker sand in the mechanical high temperature and transport properties with GGBS-based prepacked geopolymer composite. , 2023, ss.92 - 106. 10.20528/cjsmec.2023.03.002 |
AMA | ALCAN H,Aksu Alcan B,BAYRAK B,AYDIN A Potential improvement of clinker sand in the mechanical high temperature and transport properties with GGBS-based prepacked geopolymer composite. . 2023; 92 - 106. 10.20528/cjsmec.2023.03.002 |
Vancouver | ALCAN H,Aksu Alcan B,BAYRAK B,AYDIN A Potential improvement of clinker sand in the mechanical high temperature and transport properties with GGBS-based prepacked geopolymer composite. . 2023; 92 - 106. 10.20528/cjsmec.2023.03.002 |
IEEE | ALCAN H,Aksu Alcan B,BAYRAK B,AYDIN A "Potential improvement of clinker sand in the mechanical high temperature and transport properties with GGBS-based prepacked geopolymer composite." , ss.92 - 106, 2023. 10.20528/cjsmec.2023.03.002 |
ISNAD | ALCAN, HALUK GÖRKEM vd. "Potential improvement of clinker sand in the mechanical high temperature and transport properties with GGBS-based prepacked geopolymer composite". (2023), 92-106. https://doi.org/10.20528/cjsmec.2023.03.002 |
APA | ALCAN H, Aksu Alcan B, BAYRAK B, AYDIN A (2023). Potential improvement of clinker sand in the mechanical high temperature and transport properties with GGBS-based prepacked geopolymer composite. Challenge Journal of Structural Mechanics, 9(3), 92 - 106. 10.20528/cjsmec.2023.03.002 |
Chicago | ALCAN HALUK GÖRKEM,Aksu Alcan Bilge,BAYRAK Barış,AYDIN Abdulkadir Cuneyt Potential improvement of clinker sand in the mechanical high temperature and transport properties with GGBS-based prepacked geopolymer composite. Challenge Journal of Structural Mechanics 9, no.3 (2023): 92 - 106. 10.20528/cjsmec.2023.03.002 |
MLA | ALCAN HALUK GÖRKEM,Aksu Alcan Bilge,BAYRAK Barış,AYDIN Abdulkadir Cuneyt Potential improvement of clinker sand in the mechanical high temperature and transport properties with GGBS-based prepacked geopolymer composite. Challenge Journal of Structural Mechanics, vol.9, no.3, 2023, ss.92 - 106. 10.20528/cjsmec.2023.03.002 |
AMA | ALCAN H,Aksu Alcan B,BAYRAK B,AYDIN A Potential improvement of clinker sand in the mechanical high temperature and transport properties with GGBS-based prepacked geopolymer composite. Challenge Journal of Structural Mechanics. 2023; 9(3): 92 - 106. 10.20528/cjsmec.2023.03.002 |
Vancouver | ALCAN H,Aksu Alcan B,BAYRAK B,AYDIN A Potential improvement of clinker sand in the mechanical high temperature and transport properties with GGBS-based prepacked geopolymer composite. Challenge Journal of Structural Mechanics. 2023; 9(3): 92 - 106. 10.20528/cjsmec.2023.03.002 |
IEEE | ALCAN H,Aksu Alcan B,BAYRAK B,AYDIN A "Potential improvement of clinker sand in the mechanical high temperature and transport properties with GGBS-based prepacked geopolymer composite." Challenge Journal of Structural Mechanics, 9, ss.92 - 106, 2023. 10.20528/cjsmec.2023.03.002 |
ISNAD | ALCAN, HALUK GÖRKEM vd. "Potential improvement of clinker sand in the mechanical high temperature and transport properties with GGBS-based prepacked geopolymer composite". Challenge Journal of Structural Mechanics 9/3 (2023), 92-106. https://doi.org/10.20528/cjsmec.2023.03.002 |