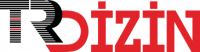
Homoprotocatechuate dioxygenase active site: Imitating the secondary sphere base via computational design
Yıl: 2023 Cilt: 47 Sayı: 5 Sayfa Aralığı: 1116 - 1124 Metin Dili: İngilizce DOI: 10.55730/1300-0527.3598 İndeks Tarihi: 21-11-2023
Homoprotocatechuate dioxygenase active site: Imitating the secondary sphere base via computational design
Öz: Oxidative ring cleavage reactions have attracted great interest and various studies on the catechol ring-cleaving enzyme homoprotocatechuate dioxygenase (HPCD) have been reported in the literature. The available data on how the proton transfer takes place led us to design a potential HPCD model structure. A secondary sphere effect of utmost importance, the assistance of His200, which is critical for the catechol proton to migrate to dioxygen, was cautiously included on the first coordination shell. This was done mainly by modifying the axial ligands in the first coordination shell of HPCD such that the dual basic/acidic role in the proton transfer pathway of His200 was reproduced. Model systems with mono-, bi-, and tridentate ligands are reported. Energetically feasible reaction channels on synthetically promising ligand structures are identified. Key structural and electronic principles for obtaining viable proton transfer paths are outlined.
Anahtar Kelime: Belge Türü: Makale Makale Türü: Araştırma Makalesi Erişim Türü: Erişime Açık
- [1] Costas M, Mehn MP, Jensen MP, Que L. Dioxygen activation at mononuclear nonheme iron active sites: enzymes, models, and intermediates. Chemical Reviews 2004; 104 (2): 939-986. https://doi.org/10.1021/cr020628n
- [2] Vaillancourt FH, Bolin JT, Eltis LD. The ins and outs of ring-cleaving dioxygenases. Critical Reviews in Biochemistry and Molecular Biology 2006; 41 (4): 241-267. https://doi.org/10.1080/10409230600817422
- [3] Solomon EI, Brunold TC, Davis MI, Kemsley JN, Lee SK et al. Geometric and electronic structure/function correlations in non- heme iron enzymes. Chemical Reviews 2000; 100 (1): 235-350. https://doi.org/10.1021/cr9900275
- [4] Blomberg MRA, Borowski T, Himo F, Liao RZ, Siegbahn PEM. Quantum chemical studies of mechanisms for metalloenzymes. Chemical Reviews 2014; 114 (7): 3601-3658. https://doi.org/10.1021/cr400388t
- [5] Broderick JB. Catechol dioxygenases. Essays in Biochemistry 1999; 34: 173-189. https://doi.org/10.1042/bse0340173
- [6] Deeth RJ, Bugg TDH. A density functional investigation of the extradiol cleavage mechanism in non-heme iron catechol dioxygenases. Journal of Biological Inorganic Chemistry 2003; 8 (4): 409-418. https://doi.org/10.1007/s00775-002-0430-7
- [7] Lipscomb JD. Mechanism of extradiol aromatic ring-cleaving dioxygenases. Current Opinion in Structural Biology 2008; 18 (6): 644-649. https://doi.org/10.1016/j.sbi.2008.11.001
- [8] Mbughuni MM, Chakrabarti M, Hayden JA, Bominaar EL, Hendrich MP et al. Trapping and spectroscopic characterization of an Fe(III)-superoxo intermediate from a nonheme mononuclear iron-containing enzyme. Proceedings of the National Academy of Sciences of the United States of America 2010; 107 (39): 16788-16793. https://doi.org/10.1073/pnas.101001510
- [9] Mbughuni MM, Chakrabarti M, Hayden JA, Meier KK, Dalluge JJ et al. Oxy intermediates of homoprotocatechuate 2,3-dioxygenase: facile electron transfer between substrates. Biochemistry 2011; 50 (47): 10262-10274. https://doi.org/10.1021/ bi201436n
- [10] Siegbahn PEM, Haeffner F. Mechanism for catechol ring-cleavage by non-heme iron extradiol dioxygenases. Journal of the American Chemical Society 2004; 126 (29): 8919-8932. https://doi.org/10.1021/ja0493805
- [11] Christian GJ, Neese F, Ye S. Unravelling the molecular origin of the regiospecificity in extradiol catechol dioxygenases. Inorganic Chemistry 2016; 55 (8): 3853-3864. https://doi.org/10.1021/acs.inorgchem.5b02978
- [12] Dong G, Shaik S, Lai W. Oxygen activation by homoprotocatechuate 2,3-dioxygenase: a QM/MM study reveals the key intermediates in the activation cycle. Chemical Science 2013; 4 (9): 3624-3635. https://doi.org/10.1039/C3SC51147B
- [13] Christian GJ, Ye S, Neese F. Oxygen activation in extradiol catecholate dioxygenases - a density functional study. Chemical Science 2012; 3 (5): 1600-1611. https://doi.org/10.1039/C2SC00754A
- [14] Groce SL, Lipscomb JD. Aromatic ring cleavage by homoprotocatechuate 2,3-dioxygenase: role of His200 in the kinetics of interconversion of reaction cycle intermediates. Biochemistry 2005; 44 (19): 7175-7188. https://doi.org/10.1021/bi050180v
- [15] Kovaleva EG, Rogers MS, Lipscomb JD. Structural basis for substrate and oxygen activation in homoprotocatechuate 2,3-dioxygenase: roles of conserved active site histidine 200. Biochemistry 2015; 54 (34): 5329-5339. https://doi.org/10.1021/acs. biochem.5b00709
- [16] Hertwig RH, Koch W. On the parameterization of the local correlation functional. What is Becke-3-LYP? Chemical Physics Letters 1997; 268 (5-6): 345-351. https://doi.org/10.1016/S0009-2614(97)00207-8
- [17] Stephens PJ, Devlin FJ, Chabalowski CF, Frisch MJ. Ab initio calculation of vibrational absorption and circular dichroism spectra using density functional force fields. Journal of Physical Chemistry 1994; 98 (45): 11623-11627. https://doi.org/10.1021/ j100096a001
- [18] Becke AD. Density-functional thermochemistry. III. The role of exact exchange. Journal of Chemical Physics 1993; 98 (7): 5648- 5652. https://doi.org/10.1063/1.464913
- [19] Lee CT, Yang WT, Parr RG. Development of the Colle-Salvetti correlation-energy formula into a functional of the electron density. Physical Review B 1988; 37 (2): 785-789. https://doi.org/10.1103/PhysRevB.37.785
- [20] Becke AD. Density-functional exchange-energy approximation with correct asymptotic behavior. Physical Review A 1988; 38 (6): 3098-3100. https://doi.org/10.1103/PhysRevA.38.3098
- [21] Vosko SH, Wilk L, Nusair M. Accurate spin-dependent electron liquid correlation energies for local spin-density calculations - a critical analysis. Canadian Journal of Physics 1980; 58 (8): 1200-1211. https://doi.org/10.1139/p80-159
- [22] Grimme S, Antony J, Ehrlich S, Krieg H. A consistent and accurate ab initio parametrization of density functional dispersion correction (DFT-D) for the 94 elements H-Pu. Journal of Chemical Physics 2010; 132 (15): 154104. http://doi. org/10.1063/1.3382344
- [23] Zhao Y, Truhlar DG. A new local density functional for main-group thermochemistry, transition metal bonding, thermochemical kinetics, and noncovalent interactions. Journal of Chemical Physics 2006; 125 (19): 194101. https://doi.org/10.1063/1.2370993
- [24] Kohn W, Becke AD, Parr RG. Density functional theory of electronic structure. Journal of Physical Chemistry 1996; 100 (31): 12974-12980. https://doi.org/10.1021/jp960669l
- [25] Parr RG, Yang W. Density Functional Theory of Atoms and Molecules. New York, NY, USA: Oxford University Press, 1989.
- [26] Dunning TH. Gaussian basis sets for use in correlated molecular calculations. I. The atoms boron through neon and hydrogen. Journal of Chemical Physics 1989; 90 (2): 1007-1023. https://doi.org/10.1063/1.456153
- [27] Fukui K. The path of chemical reactions - the IRC approach. Accounts of Chemical Research 1981; 14 (12): 363-368. https://doi. org/10.1021/ar00072a001
- [28] Frisch MJ, Trucks GW, Schlegel HB, Scuseria GE, Robb MA et al. Gaussian 09. Wallingford, CT, USA: Gaussian, Inc., 2009.
- [29] Dong G, Lai W. Reaction mechanism of homoprotocatechuate 2,3-dioxygenase with 4-nitrocatechol: implications for the role of substrate. Journal of Physical Chemistry B 2014; 118 (7): 1791-1798. https://doi.org/10.1021/jp411812m
- [30] Yalçın S. Quantum chemical investigation of O-O and C-C bond activation reactions of homoprotocatechuate 2,3-dioxygenase active site complex. MSc, Gazi University, Ankara, Türkiye, 2015 (in Turkish).
- [31] Poulos TL. Heme enzyme structure and function. Chemical Reviews 2014; 114 (7): 3919-3962. https://doi.org/10.1021/cr400415k
- [32] Sanvoisin J, Langley GJ, Bugg TDH. Mechanism of extradiol catechol dioxygenases: evidence for a lactone intermediate in the 2,3-dihydroxyphenylpropionate 1,2-dioxygenase reaction. Journal of the American Chemical Society 1995; 117 (29): 7836-7837. https://doi.org/10.1021/ja00134a041
- [33] Chatterjee S, Sheet D, Paine TK. Catalytic and regiospecific extradiol cleavage of catechol by a biomimetic iron complex. Chemical Communications 2013; 49 (87): 10251-10253. https://doi.org/10.1039/C3CC44124E
- [34] Kendall AJ, Zakharov LN, Gilbertson JD. Synthesis and stabilization of a monomeric iron(II) hydroxo complex via intramolecular hydrogen bonding in the secondary coordination sphere. Inorganic Chemistry 2010; 49 (19): 8656-8658. https:// doi.org/10.1021/ic101408e
- [35] Shook RL, Borovik AS. Role of the secondary coordination sphere in metal-mediated dioxygen activation. Inorganic Chemistry 2010; 49 (8): 3646-3660. https://doi.org/10.1021/ic901550k
- [36] Nagaraju P, Ohta T, Liu JG, Ogura T, Naruta Y. The secondary coordination sphere controlled reactivity of a ferric-superoxo heme: unexpected conversion to a ferric hydroperoxo intermediate by reaction with a high-spin ferrous heme. Chemical Communications 2016; 52 (45): 7213-7216. https://doi.org/10.1039/C6CC02162J
- [37] Sahu S, Goldberg DP. Activation of dioxygen by iron and manganese complexes: a heme and nonheme perspective. Journal of the American Chemical Society 2016; 138 (36): 11410-11428. https://doi.org/10.1021/jacs.6b05251
- [38] Sahu S, Widger LR, Quesne MG, de Visser SP, Matsumura H et al. Secondary coordination sphere influence on the reactivity of nonheme iron(II) complexes: an experimental and DFT approach. Journal of the American Chemical Society 2013; 135 (29): 10590-10593. https://doi.org/10.1021/ja402688t
- [39] Ohta T, Nagaraju P, Liu JG, Ogura T, Naruta Y. The secondary coordination sphere and axial ligand effects on oxygen reduction reaction by iron porphyrins: a DFT computational study. Journal of Biological Inorganic Chemistry 2016; 21 (5): 745-755. https://doi.org/10.1007/s00775-016-1380-9
- [40] Bansal D, Kumar G, Hundal G, Gupta R. Mononuclear complexes of amide-based ligands containing appended functional groups: role of secondary coordination spheres on catalysis. Dalton Transactions 2014; 43 (39): 14865-14875. https://doi. org/10.1039/C4DT02079K
- [41] Büyüktemiz M. Active center design of non-heme enzymes based on secondary sphere effects. MSc, Gazi University, Ankara, Türkiye, 2020 (in Turkish).
APA | Buyuktemiz M, Dede Y (2023). Homoprotocatechuate dioxygenase active site: Imitating the secondary sphere base via computational design. , 1116 - 1124. 10.55730/1300-0527.3598 |
Chicago | Buyuktemiz Muhammed,Dede Yavuz Homoprotocatechuate dioxygenase active site: Imitating the secondary sphere base via computational design. (2023): 1116 - 1124. 10.55730/1300-0527.3598 |
MLA | Buyuktemiz Muhammed,Dede Yavuz Homoprotocatechuate dioxygenase active site: Imitating the secondary sphere base via computational design. , 2023, ss.1116 - 1124. 10.55730/1300-0527.3598 |
AMA | Buyuktemiz M,Dede Y Homoprotocatechuate dioxygenase active site: Imitating the secondary sphere base via computational design. . 2023; 1116 - 1124. 10.55730/1300-0527.3598 |
Vancouver | Buyuktemiz M,Dede Y Homoprotocatechuate dioxygenase active site: Imitating the secondary sphere base via computational design. . 2023; 1116 - 1124. 10.55730/1300-0527.3598 |
IEEE | Buyuktemiz M,Dede Y "Homoprotocatechuate dioxygenase active site: Imitating the secondary sphere base via computational design." , ss.1116 - 1124, 2023. 10.55730/1300-0527.3598 |
ISNAD | Buyuktemiz, Muhammed - Dede, Yavuz. "Homoprotocatechuate dioxygenase active site: Imitating the secondary sphere base via computational design". (2023), 1116-1124. https://doi.org/10.55730/1300-0527.3598 |
APA | Buyuktemiz M, Dede Y (2023). Homoprotocatechuate dioxygenase active site: Imitating the secondary sphere base via computational design. Turkish Journal of Chemistry, 47(5), 1116 - 1124. 10.55730/1300-0527.3598 |
Chicago | Buyuktemiz Muhammed,Dede Yavuz Homoprotocatechuate dioxygenase active site: Imitating the secondary sphere base via computational design. Turkish Journal of Chemistry 47, no.5 (2023): 1116 - 1124. 10.55730/1300-0527.3598 |
MLA | Buyuktemiz Muhammed,Dede Yavuz Homoprotocatechuate dioxygenase active site: Imitating the secondary sphere base via computational design. Turkish Journal of Chemistry, vol.47, no.5, 2023, ss.1116 - 1124. 10.55730/1300-0527.3598 |
AMA | Buyuktemiz M,Dede Y Homoprotocatechuate dioxygenase active site: Imitating the secondary sphere base via computational design. Turkish Journal of Chemistry. 2023; 47(5): 1116 - 1124. 10.55730/1300-0527.3598 |
Vancouver | Buyuktemiz M,Dede Y Homoprotocatechuate dioxygenase active site: Imitating the secondary sphere base via computational design. Turkish Journal of Chemistry. 2023; 47(5): 1116 - 1124. 10.55730/1300-0527.3598 |
IEEE | Buyuktemiz M,Dede Y "Homoprotocatechuate dioxygenase active site: Imitating the secondary sphere base via computational design." Turkish Journal of Chemistry, 47, ss.1116 - 1124, 2023. 10.55730/1300-0527.3598 |
ISNAD | Buyuktemiz, Muhammed - Dede, Yavuz. "Homoprotocatechuate dioxygenase active site: Imitating the secondary sphere base via computational design". Turkish Journal of Chemistry 47/5 (2023), 1116-1124. https://doi.org/10.55730/1300-0527.3598 |