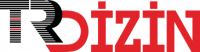
Architecture design of $TiO_2$ with Co-doped CdS quantum dots photoelectrode for water splitting
Yıl: 2023 Cilt: 47 Sayı: 5 Sayfa Aralığı: 1183 - 1194 Metin Dili: İngilizce DOI: 10.55730/1300-0527.3604 İndeks Tarihi: 21-11-2023
Architecture design of $TiO_2$ with Co-doped CdS quantum dots photoelectrode for water splitting
Öz: Photoelectrochemical hydrogen production is a critical key to solving the carbon-zero goal of countries due to renewable sources of solar light and combustion products of hydrogen-only water. Here, an architecture design for an n-type nano rosettes-rod $TiO_2$ (RT) surface using CdS and Co-doped CdS quantum dots (QDs) is carried out utilizing the SILAR (simple ionic layer adsorption and reaction) method. Furthermore, the photocatalytic behaviour of Co-doped CdS QDs SILAR cycles deposition is investigated in various cycles, including 5, 8, 10, and 12. The FESEM, Raman XRD, Uv-Vis spectrometer, and vibration modes are used to evaluate the photoelectrode surface structure, crystal structure, and solar light absorption, respectively. FESEM images and XRD pattern revealed successive CdS QDS and Co-doped CdS QDs deposition on the RT boundary and rising SILAR cycles of Co-doped CdS QDs lead to further coverage of RT surface. UV-vis spectrometer indicated shifting solar light absorption to the visible region by applying more SILAR cycles of Co-doped CdS QDs deposition. The electrochemical parameters obtained from EIS showed total polarization resistance $(R_p)$ of the RT electrode dramatically decreased with 10 SILAR cycle Co-doped CdS QDs deposition $(5093 Ω cm^2 and 617 Ω cm^2)$. Linear sweep voltammetry (LSV) and chronoamperometric photocatalytic performance measurements indicated Co-doped CdS QDs on RT extremely enhanced photoresponse under solar irradiation and 10 SILAR cycle Co-doped CdS QDs improved photocurrent density about fourfold according to blank RT electrode.
Anahtar Kelime: Belge Türü: Makale Makale Türü: Araştırma Makalesi Erişim Türü: Erişime Açık
- [1] Simpson NP, Mach KJ, Constable A, Hess J, Hogarth R et al. A framework for complex climate change risk assessment. One Earth 2021; 4 (4): 489-501. https://doi.org/10.1016/j.oneear.2021.03.005
- [2] Valentová A, Bostik V. Climate change and human health. Military Medical Science Letters 2021; 90 (2): 50. https://doi.org/10.31482/ mmsl.2021.010
- [3] Short JR, Farmer A. Cities and Climate Change. Earth (Switzerland) 2021; 2 (4): 1038-1045. https://doi.org/10.3390/earth2040061
- [4] Heffernan E, Pan W, Liang X, de Wilde P. Zero carbon homes: Perceptions from the UK construction industry. Energy Policy 2015; 79: 23-36. https://doi.org/10.1016/j.enpol.2015.01.005
- [5] Seto KC, Churkina G, Hsu A, Keller M, Newman PWG et al. From Low- To Net-Zero Carbon Cities- To Next Global Agenda. Annual Review of Environment and Resources 2021; 46: 377-415. https://doi.org/10.1146/annurev-environ-050120-113117
- [6] Li X, Zhao L, Yu J, Liu X, Zhang X et al. Water Splitting: From Electrode to Green Energy System. Nano-Micro Letters 2020; 12: 1-29. https://doi.org/10.1007/s40820-020-00469-3
- [7] Joy J, Mathew J, George SC. Nanomaterials for photoelectrochemical water splitting–review. International Journal of Hydrogen Energy, 2018; 43 (10): 4804-4817. https://doi.org/10.1016/j.ijhydene.2018.01.099
- [8] Mohsin M, Ishaq T, Bhatti IA, Maryam, Jilani A et al. Semiconductor Nanomaterial Photocatalysts for Water-Splitting Hydrogen Production: The Holy Grail of Converting Solar Energy to Fuel. Nanomaterials 2023; 13 (3): 546. https://doi.org/10.3390/nano13030546
- [9] Hota P, Das A, Maiti DK. A short review on generation of green fuel hydrogen through water splitting. International Journal of Hydrogen Energy 2023; 48 (2): 523-541. https://doi.org/10.1016/j.ijhydene.2022.09.264
- [10] Galdámez-Martinez A, Santana G, Güell F, Martínez-Alanis PR, Dutt A. Photoluminescence of ZnO nanowires: A review. Nanomaterials 2020; 10 (5): 857. https://doi.org/10.3390/nano10050857
- [11] Albiter E, Merlano AS, Rojas E, Barrera-Andrade JM, Salazar Á et al. Synthesis, characterization, and photocatalytic performance of ZnO– graphene nanocomposites: A review. Journal of Composites Science 2021; 5 (1): 4. https://doi.org/10.3390/jcs5010004
- [12] Tran VA, Nguyen TP, Le VT, Kim IT, Lee SW et al. Excellent photocatalytic activity of ternary Ag@WO3@rGO nanocomposites under solar simulation irradiation. Journal of Science: Advanced Materials and Devices 2021; 6 (1): 108-117. https://doi.org/10.1016/j. jsamd.2020.12.001
- [13] TayebiM,MasoumiZ,LeeBK.UltrasonicallypreparedphotocatalystofW/WO3 nanoplateswithWS2 nanosheetsas2Dmaterialforimproving photoelectrochemical water splitting. Ultrasonics Sonochemistry 2021; 70: 105339. https://doi.org/10.1016/j.ultsonch.2020.105339
- [14] Yu R, Chen Q, Li W, Yu X, Zhong Q. Decorating Cu2O with Ni-doped metal organic frameworks as efficient photocathodes for solar water splitting. International Journal of Hydrogen Energy 2023; 48 (45): 17065-17073. doi.org/10.1016/j.ijhydene.2023.01.145
- [15] Yang L, Wang R, Chu D, Chen Z, Zhong F et al. $BiVO_4$ photoelectrodes for unbiased solar water splitting devices enabled by electrodepositing of Cu2O simultaneously as photoanode and photocathode. Journal of Alloys and Compounds 2023; 945: 169336. https://doi.org/10.1016/j. jallcom.2023.169336
- [16] Siavash Moakhar R, Hosseini-Hosseinabad SM, Masudy-Panah S, Seza A, Jalali M et al. Photoelectrochemical Water-Splitting Using CuO- Based Electrodes for Hydrogen Production: A Review. Advanced Materials 2021; 33 (33): 2007285. https://doi.org/10.1002/adma.202007285
- [17] de Brito JF, Tavella F, Genovese C, Ampelli C, Zanoni MVB et al. Role of CuO in the modification of the photocatalytic water splitting behavior of TiO2 nanotube thin films. Applied Catalysis B: Environmental 2018; 224: 136-145. https://doi.org/10.1016/j.apcatb.2017.09.071
- [18] Baran Aydın E, Ateş S, Sığırcık G. $CuO-TiO_2$ nanostructures prepared by chemical and electrochemical methods as photo electrode for hydrogen production. International Journal of Hydrogen Energy 2022; 47.10: 6519-6534. https://doi.org/10.1016/j.ijhydene.2021.12.032
- [19] Anantha Prabhu C, Silambarasan D, Sarika R, Selvam V. Synthesis and characterization of $TiO_2$. Materials Today: Proceedings 2022; 64: 1793-1797. https://doi.org/10.1016/j.matpr.2022.06.074
- [20] Gupta SM, Tripathi M. A review of $TiO_2$ nanoparticles. Chinese Science Bulletin 2011; 56 (16): 1639-1657. https://doi.org/10.1007/s11434- 011-4476-1
- [21] Li B, Wu S, Gao X. Theoretical calculation of a $TiO_2$-based photocatalyst in the field of water splitting: A review. Nanotechnology Reviews 2020; 9 (1): 1080-1103. https://doi.org/10.1515/ntrev-2020-0085
- [22] Aydin EB, Siğircik G, Takci HAM. Antimicrobial properties and corrosion behavior of TiO2–NTs electrodes modified with Ag and ZnO nanorod in simulated body fluid solution. Journal of Molecular Structure 2021; 1240: 130569. https://doi.org/10.1016/j.molstruc.2021.130569
- [23] Zhang X, Zhang S, Cui X, Zhou W, Cao W et al. Recent Advances in TiO2-based Photoanodes for Photoelectrochemical Water Splitting. Chemistry–An Asian Journal 2022; 17 (20): 202200668. https://doi.org/10.1002/asia.202200668
- [24] Zallen R, Moret MP. The optical absorption edge of brookite TiO2. Solid State Communications, 2006; 137 (3): 154-157. https://doi. org/10.1016/j.ssc.2005.10.024
- [25] Liu H, Li D, Yang X, Li H. Fabrication and characterization of $Ag_3PO_4/TiO_2$ heterostructure with improved visible-light photocatalytic activity for the degradation of methyl orange and sterilization of E.coli. Materials Technology 2019; 34 (4): 192-203. https://doi.org/10.108 0/10667857.2018.1545391
- [26] Ücker CL, Riemke F, Goetzke V, Moreira ML, Raubach CW et al. Facile preparation of $Nb_2O_5/TiO_2$ heterostructures for photocatalytic application. Chemical Physics Impact 2022; 4: 100079. https://doi.org/10.1016/j.chphi.2022.100079
- [27] My Tran N, Thanh Hoai Ta Q, Noh JS. Unusual synthesis of safflower-shaped $TiO2/Ti_3C_2$ heterostructures initiated from two-dimensional Ti3C2 MXene. Applied Surface Science 2021; 538: 148023. https://doi.org/10.1016/j.apsusc.2020.148023
- [28] Nandi P, Das D. ZnO/CdS/CuS heterostructure: A suitable candidate for applications in visible-light photocatalysis. Journal of Physics and Chemistry of Solids 2022; 160: 110344. https://doi.org/10.1016/j.jpcs.2021.110344
- [29] Jia Y, Zhang Y, Wei X, Guo T, Fan J et al. GaTe/CdS heterostructure with tunable electronic properties via external electric field and biaxial strain. Journal of Alloys and Compounds 2020; 832: 154965. https://doi.org/10.1016/j.jallcom.2020.154965
- [30] Guo X, Liu X, Yan J, Liu S. Heteroepitaxial growth of core-shell ZnO/CdS heterostructure for efficient and stable photocatalytic hydrogen generation. International Journal of Hydrogen Energy 2022; 47 (81): 34410-34420. https://doi.org/10.1016/j.ijhydene.2022.08.032
- [31] Sun WT, Yu A, Pan HY, Gao XF, Chen Q et al. CdS quantum dots sensitized $TiO_2$ nanotube-array photoelectrodes. Journal of the American Chemical Society 2008; 130: 1124-1125. https://doi.org/10.1021/ja0777741
- [32] Sivaranjani K, Sivakumar S, Dharmaraja J. Enhancement Photocatalytic Activity of Mn Doped CdS/ZnO Nanocomposites for the Degradation of Methylene Blue Under Solar Light Irradiation. Advances in Materials Science 2022; 22 (2): 28-48. https://doi.org/10.2478/ adms-2022-0006
- [33] Muruganandam S, Anbalagan G, Murugadoss G. Optical and magnetic properties of PVP surfactant with Cu doped CdS nanoparticles. Optik 2017; 130: 82-90. https://doi.org/10.1016/j.ijleo.2016.11.053
- [34] Diaz-Grijalva OI, Berman-Mendoza D, Flores-Pacheco A, López-Delgado R, Ramos-Carrazco A et al. Cu-doped CdS thin films by chemical bath deposition and ion exchange. Journal of Materials Science: Materials in Electronics 2020; 31: 1722-1730. https://doi.org/10.1007/ s10854-019-02690-2
- [35] Ibraheem F, Mahdy MA, Mahmoud EA, Ortega JE, Rogero C et al. Tuning Paramagnetic effect of Co-doped CdS diluted magnetic semiconductor quantum dots. Journal of Alloys and Compounds 2020; 834: 155196. https://doi.org/10.1016/j.jallcom.2020.155196
- [36] Gbashi KR, Muhi MAH, Jabbar AA, Mahmood NB, Hasan RF. Copper dopants impact enhanced behavior of Mn:Cu co-doped CdS nanocrystals (quantum dots) and their characteristics for optoelectronic applications. Applied Physics A Process 2020; 126.8: 628. https:// doi.org/10.1007/s00339-020-03801-1
- [37] Ahmad A, Tezcan F, Yerlikaya G, Zia-ur-Rehman, Paksoy Het al. Solar light driven photoelectrochemical water splitting using Mn-doped CdS quantum dots sensitized hierarchical rosette-rod TiO2 photoanodes. Journal of Electroanalytical Chemistry 2022; 916: 116384. https:// doi.org/10.1016/j.jelechem.2022.116384
- [38] Ganguly A, Nath SS. Mn-doped CdS quantum dots as sensitizers in solar cells. Materials Science and Engineering: B 2020; 255: 114532. https://doi.org/10.1016/j.mseb.2020.114532 TEZCAN et al. / Turk J Chem1194
- [39] Zou X, He S, Teng G, Zhao C. Performance study of CdS/Co-doped-CdSe quantum dot sensitized solar cells. Journal of Nanomaterials 2014; 2014: 50-50. https://doi.org/10.1155/2014/818160
- [40] Meng X, Zhang C, Dong C, Sun W, Ji D et al. Carbon quantum dots assisted strategy to synthesize Co@NC for boosting photocatalytic hydrogen evolution performance of CdS. Chemical Engineering Journal 2020; 389: 124432. https://doi.org/10.1016/j.cej.2020.124432
- [41] Thambidurai M, Muthukumarasamy N, Velauthapillai D, Agilan S, Balasundaraprabhu R. Structural, optical, and electrical properties of cobalt-doped CdS quantum dots. Journal of Electronic Materials 2012; 41: 665-672. https://doi.org/10.1007/s11664-012-1900-5
- [42] Liu B, Aydil ES. Growth of oriented single-crystalline rutile $TiO_2$ nanorods on transparent conducting substrates for dye-sensitized solar cells. Advanced Energy Conversion 2009; 3 (2), 455-479. https://doi.org/10.1021/ja8078972
- [43] Chen J, Song W, Hou H, Zhang Y, Jing M et al. Ti3+ Self-Doped Dark Rutile $TiO_2$ Ultrafine Nanorods with Durable High-Rate Capability for Lithium-Ion Batteries. Advanced Functional Materials2015; 25 (43); 6793-6801. https://doi.org/10.1002/adfm.201502978
- [44] Chen H, Fu W, Yang H, Sun P, Zhang Y et al. Photosensitization of $TiO_2$ nanorods with CdS quantum dots for photovoltaic devices. Electrochimica Acta 2010; 56 (2), 919-924. https://doi.org/10.1016/j.electacta.2010.10.003
- [45] Pan Z, Qiu Y, Yang J, Liu M, Zhou L et al. Synthesis of three-dimensional hyperbranched $TiO_2$ nanowire arrays with significantly enhanced photoelectrochemical hydrogen production. Journal of Materials Chemistry A, 2015; 3 (7): 4004-4009. https://doi.org/10.1039/c4ta06498d
- [46] Muthalif MPA, Lee YS, Sunesh CD, Kim HJ, Choe Y. Enhanced photovoltaic performance of quantum dot-sensitized solar cells with a progressive reduction of recombination using Cu-doped CdS quantum dots. Applied Surface Science 2017; 2 (396): 582-589. https://doi. org/10.1016/j.apsusc.2016.10.200
- [47] Gopi CVVM, Venkata-Haritha M, Kim SK, Kim HJ. A strategy to improve the energy conversion efficiency and stability of quantum dot-sensitized solar cells using manganese-doped cadmium sulfide quantum dots. Dalton Transactions 2015; 44 (2): 630-638. https://doi. org/10.1039/c4dt03063j
- [48] Janbandhu SY, Munishwar SR, Sukhadeve GK, Gedam RS. Effect of annealing time on optical properties of CdS QDs containing glasses and their application for degradation of methyl orange dye. Materials Characterization 2019; 152: 230-238. https://doi.org/10.1016/j. matchar.2019.04.027
- [49] Yadav AN, Kumar R, Jaiswal RK, Singh AK, Kumar P et al. Surface modification of CdS quantum dots: An effective approach for improving biocompatibility. Materials Research Express 2019; 6 (5): 055002. https://doi.org/10.1088/2053-1591/aaff70
- [50] Gao X, Liu X, Zhu Z, Wang X, Xie Z. Enhanced photoelectrochemical and photocatalytic behaviors of $MFe_2O_4$ (M = Ni, Co, Zn and Sr) modified $TiO_2$ nanorod arrays. Scientific Reports 2016; 6 (1): 30543. https://doi.org/10.1038/srep30543
- [51] Schreder B, Dem C, Schmitt M, Materny A, Kiefer W et al. Raman spectroscopy of II-VI semiconductor nanostructures: CdS quantum dots. Journal of Raman Spectroscopy 2003; 34 (2): 100-103. https://doi.org/10.1002/jrs.959
- [52] Nel JM, Gaigher HL, Auret FD. Microstructures of electrodeposited CdS layers. Thin Solid Films 2003; 436 (2): 186-195. https://doi. org/10.1016/S0040-6090(03)00570-4
- [53] Bendjama M, Hamdaoui O, Ferkous H, Alghyamah A. Removal of Safranin O from Water by $UV/TiO_2/IO_3$− Advanced Oxidation Process: Parametric Study and Impact of Inorganic Ions and Humic Acid. Catalysts 2023; 13 (2): 447. https://doi.org/10.3390/catal13020447
- [54] Muruganandam S, Parivathini K, Murugadoss G. Effect of co-doped $(Ni^{2+}:Co^{2+})$ in CdS nanoparticles: investigation on structural and magnetic properties. Applied Physics A 2021; 127 (6): 400. https://doi.org/10.1007/s00339-021-04555-0
- [55] Murugesan R, Sivakumar S, Karthik K, Anandan P, Haris M. Effect of Mg/Co on the properties of CdS thin films deposited by spray pyrolysis technique. Current Applied Physics 2019; 19 (10): 1136-1144. https://doi.org/10.1016/j.cap.2019.07.008
- [56] Shah SA, Khan I, Yuan A. MoS2 as a Co-Catalyst for Photocatalytic Hydrogen Production: A Mini Review. Molecules 2022; 27 (10): 3289. https://doi.org/10.3390/molecules27103289
- [57] Huerta-Flores AM, Chávez-Angulo G, Carrasco-Jaim OA, Torres-Martínez LM, Garza-Navarro MA. Enhanced photoelectrochemical water splitting on heterostructured $α-Fe_2O_3-TiO_2:X$ (X = Co, Cu, Bi) photoanodes: Role of metal doping on charge carrier dynamics improvement. Journal of Photochemistry and Photobiology A: Chemistry 2021; 410: 113077. https://doi.org/10.1016/j.jphotochem.2020.113077
- [58] Dey KK, Gahlawat S, Ingole PP. $BiVO_4$ optimized to nano-worm morphology for enhanced activity towards photoelectrochemical water splitting. Journal of Materials Chemistry A 2019; 7 (37): 21207-21221. https://doi.org/10.1039/c9ta07353a
- [59] Braiek Z, Ben Naceur J, Jrad F, Ben Assaker I, Chtourou R. Novel synthesis of graphene oxide/In2S3/TiO2 NRs heterojunction photoanode for enhanced photoelectrochemical (PEC) performance. International Journal of Hydrogen Energy 2022; 47 (6): 3655-3666. https://doi. org/10.1016/j.ijhydene.2021.10.268
- [60] Gao XF, Sun WT, Ai G, Peng LM. Photoelectric performance of $TiO_2$ nanotube array photoelectrodes cosensitized with CdS/CdSe quantum dots. Applied Physics Letters 2010; 96 (15): 153104. https://doi.org/10.1063/1.3386525
- [61] Orimolade BO, Koiki BA, Peleyeju GM, Arotiba OA. Visible light driven photoelectrocatalysis on a $FTO/BiVO_4/BiOI$ anode for water treatment involving emerging pharmaceutical pollutants. Electrochimica Acta 2019; 307: 285-292. https://doi.org/10.1016/j. electacta.2019.03.217
APA | tezcan f, Ahmad A, Kardaş G (2023). Architecture design of $TiO_2$ with Co-doped CdS quantum dots photoelectrode for water splitting. , 1183 - 1194. 10.55730/1300-0527.3604 |
Chicago | tezcan fatih,Ahmad Abrar,Kardaş Gülfeza Architecture design of $TiO_2$ with Co-doped CdS quantum dots photoelectrode for water splitting. (2023): 1183 - 1194. 10.55730/1300-0527.3604 |
MLA | tezcan fatih,Ahmad Abrar,Kardaş Gülfeza Architecture design of $TiO_2$ with Co-doped CdS quantum dots photoelectrode for water splitting. , 2023, ss.1183 - 1194. 10.55730/1300-0527.3604 |
AMA | tezcan f,Ahmad A,Kardaş G Architecture design of $TiO_2$ with Co-doped CdS quantum dots photoelectrode for water splitting. . 2023; 1183 - 1194. 10.55730/1300-0527.3604 |
Vancouver | tezcan f,Ahmad A,Kardaş G Architecture design of $TiO_2$ with Co-doped CdS quantum dots photoelectrode for water splitting. . 2023; 1183 - 1194. 10.55730/1300-0527.3604 |
IEEE | tezcan f,Ahmad A,Kardaş G "Architecture design of $TiO_2$ with Co-doped CdS quantum dots photoelectrode for water splitting." , ss.1183 - 1194, 2023. 10.55730/1300-0527.3604 |
ISNAD | tezcan, fatih vd. "Architecture design of $TiO_2$ with Co-doped CdS quantum dots photoelectrode for water splitting". (2023), 1183-1194. https://doi.org/10.55730/1300-0527.3604 |
APA | tezcan f, Ahmad A, Kardaş G (2023). Architecture design of $TiO_2$ with Co-doped CdS quantum dots photoelectrode for water splitting. Turkish Journal of Chemistry, 47(5), 1183 - 1194. 10.55730/1300-0527.3604 |
Chicago | tezcan fatih,Ahmad Abrar,Kardaş Gülfeza Architecture design of $TiO_2$ with Co-doped CdS quantum dots photoelectrode for water splitting. Turkish Journal of Chemistry 47, no.5 (2023): 1183 - 1194. 10.55730/1300-0527.3604 |
MLA | tezcan fatih,Ahmad Abrar,Kardaş Gülfeza Architecture design of $TiO_2$ with Co-doped CdS quantum dots photoelectrode for water splitting. Turkish Journal of Chemistry, vol.47, no.5, 2023, ss.1183 - 1194. 10.55730/1300-0527.3604 |
AMA | tezcan f,Ahmad A,Kardaş G Architecture design of $TiO_2$ with Co-doped CdS quantum dots photoelectrode for water splitting. Turkish Journal of Chemistry. 2023; 47(5): 1183 - 1194. 10.55730/1300-0527.3604 |
Vancouver | tezcan f,Ahmad A,Kardaş G Architecture design of $TiO_2$ with Co-doped CdS quantum dots photoelectrode for water splitting. Turkish Journal of Chemistry. 2023; 47(5): 1183 - 1194. 10.55730/1300-0527.3604 |
IEEE | tezcan f,Ahmad A,Kardaş G "Architecture design of $TiO_2$ with Co-doped CdS quantum dots photoelectrode for water splitting." Turkish Journal of Chemistry, 47, ss.1183 - 1194, 2023. 10.55730/1300-0527.3604 |
ISNAD | tezcan, fatih vd. "Architecture design of $TiO_2$ with Co-doped CdS quantum dots photoelectrode for water splitting". Turkish Journal of Chemistry 47/5 (2023), 1183-1194. https://doi.org/10.55730/1300-0527.3604 |