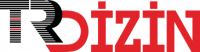
Etkileşen Sistemler ile Kuantum Termometreler
Proje Grubu: MFAG Sayfa Sayısı: 64 Proje No: 122F371 Proje Bitiş Tarihi: 15.11.2023 Metin Dili: Türkçe DOI: 122F371 İndeks Tarihi: 04-03-2024
Etkileşen Sistemler ile Kuantum Termometreler
Öz: Bu proje, ölçüm hassasiyetini artırmayı ve uygulanabilir sıcaklık aralığını genişletmeyi amaçlayan düşük sıcaklık termometri şemalarını sunmayı hedeflemektedir. Özellikle, kuantum termometrelerini sistemli bir şekilde incelemeyi, sıcaklık ölçümlerindeki temel sınırlarını belirlemeyi ve kuantum koherans (quantum coherence) ve kuantum dolanıklık (entanglement) gibi özelliklerle donatarak geliştirmeyi amaçlıyoruz. Ayrıca, termal koherant bir durumu (thermal coherent state) hazırlamak için teorik bir model öneriyoruz ve bunun kuantum termometrisinde etkinliğini değerlendiriyoruz. Son olarak, bir optomekanik kuantum sisteminden yararlanarak kuantum termometrisi için daha uygulanabilir ve pratik bir teknik üzerinde araştırma yapıyoruz. Son yıllarda, düşük sıcaklık kuantum termometrisini iyileştirmek için birçok öneri ortaya çıktı. Tipik bir sıcaklık ölçümünde beklenti, probun ölçülmek istenen örnek ile termal dengeye geçmesidir. Belirli bir enerji spektrumuna sahip problar, termal denge durumundaki tek bir sıcaklık için uygundur. Bir dizi sıcaklık için optimal algılama elde etmek, probun daha yüksek uyarılmış durumlarında yüksek derecede dejenere veya harici periyodik uyarılma içeren komplike kontrol yöntemleri gerektirir. Mevcut öneriler yeni nesil kuantum koherans, süperpozisyon, dolanıklık gibi kuantum durumlarının avantajlarından istifade eden, kuantum termometreler için ideal değildir. Yeni nesil kuantum avantajları kullanabilen ve geniş bir düşük sıcaklık aralığını ölçeebilen bir kuantum termal probu tanımlamak arzu edilmektedir. Ayrıca, kuantum termometrisi için mevcut fiziksel düzenekleri, örneğin kubit-rezonatör ve saf optomekanik sistemler gibi, deneysel gerçekleme potansiyellerini göstermek kritiktir. Bu sistemler, düşük sıcaklıkta hassas ölçümler yapma yeteneğiini korurken sıcaklık tahmininin pratik uygulanabilir aralığıını genişletir. Bu zorlukları ele almak için projemiz, koherans (coherence), termal koherant durumlar (thermal coherent state) ve enerji seviyelerindeki parametre bağımlı dejenerasyonlarının sıcaklık tahminine olan etkisini daha derinlemesine anlamak için basit modeller sunmaktadır
Anahtar Kelime: Quantum Thermometers with Interacting Systems
Öz: This project aims to present low-temperature thermometry schemes to enhance measurement precision and broaden the range. In particular, we aim to systematically examine quantum thermometers, determine their fundamental limits in temperature measurements, and develop them with features such as quantum coherence and entanglement. In addition, we also present a theoretical model for preparing a thermal coherent state and investigate its efficacy in quantum thermometry. Finally, we investigate a feasible and more practical technique for quantum thermometry with the help of an optomechanical quantum system. In recent years, numerous proposals have emerged to improve low-temperature quantum thermometry. In a typical temperature measurement setup, the expectation is for the probe to undergo thermalization with the sample to be measured. Probes with a specific energy spectrum are optimal for a single temperature at thermal equilibrium. Achieving optimal sensing for a range of temperatures requires high degeneracy in the higher excited states of the probe or a non-autonomous scheme involving external periodic driving. However, these proposals must explore the impact of the probe?s genuine quantum features, such as quantum coherence. The challenge remains to identify a probe capable of utilizing quantum features to measure a broad temperature range. Moreover, it is crucial to present readily available physical setups, such as qubit-resonator and pure optomechanical systems, for quantum thermometry, demonstrating their potential feasibility for experimental realization. These systems retain the same capability to achieve precise measurements at low-temperature while broadening the applicable range of temperature estimation. In tackling these challenges, our project introduces simple models to provide a more profound understanding of the impact of coherence, thermal coherent states, and parameter-dependent degeneracy in energy levels on temperature estimation.
Anahtar Kelime: Erişim Türü: Erişime Açık
- Abari, N. E., Rakhubovsky, A. A., & Filip, R. 2022. “Thermally-induced qubit coherence in quantum electromechanics”. New J. Phys., 24(11), 113006.
- Abiuso, P., Erdman, P. A., Ronen, M., Noé, F., Haack, G., & Perarnau-Llobet, M. ???? “Optimal Ther- mometers with Spin Networks”.
- Alves, G. O., & Landi, G. T. 2022. “Bayesian estimation for collisional thermometry”. Phys. Rev. A, 105, 012212.
- Anto-Sztrikacs, N., Miller, H. J. D., Nazir, A., & Segal, D. 2023. “Bypassing thermalization timescales in temperature estimation using prethermal probes”.
- Aspelmeyer, M., Kippenberg, T. J., & Marquardt, F. 2014. “Cavity optomechanics”. Rev. Mod. Phys., 86, 1391–1452.
- Bennett, S. D., Yao, N. Y., Otterbach, J., Zoller, P., Rabl, P., & Lukin, M. D. 2013. “Phonon-Induced Spin-Spin Interactions in Diamond Nanostructures: Application to Spin Squeezing”. Phys. Rev. Lett., 110, 156402.
- Binder, F., Correa, L. A., Gogolin, C., Anders, J., & Adesso, G. 2018. “Thermodynamics in the quantum regime”. Fundamental Theories of Physics, 195, 1–2.
- Bishop, R. F., & Vourdas, A. 1987. “Coherent mixed states and a generalised P representation”. J. Phys. A: Math. Gen., 20(12), 3743.
- Blais, A., Huang, R.-S., Wallraff, A., Girvin, S. M., & Schoelkopf, R. J. 2004. “Cavity quantum electrody- namics for superconducting electrical circuits: An architecture for quantum computation”. Phys. Rev. A, 69, 062320.
- Bloch, I., Dalibard, J., & Nascimbène, S. 2012. “Quantum simulations with ultracold quantum gases”. Nature Phys., 8(4), 267–276.
- Bloch, I., Dalibard, J., & Zwerger, W. 2008. “Many-body physics with ultracold gases”. Rev. Mod. Phys., 80, 885–964.
- Boeyens, J., Seah, S., & Nimmrichter, S. 2021. “Uninformed Bayesian quantum thermometry”. Phys. Rev. A, 104, 052214.
- Bouton, Q., Nettersheim, J., Adam, D., Schmidt, F., Mayer, D., Lausch, T., Tiemann, E., & Widera, A. 2020. “Single-Atom Quantum Probes for Ultracold Gases Boosted by Nonequilibrium Spin Dynamics”. Phys. Rev. X, 10, 011018.
- Braunstein, S. L., & Caves, C. M. 1994. “Statistical distance and the geometry of quantum states”. Phys. Rev. Lett., 72, 3439–3443.
- Breuer, H.-P., & Petruccione, F. 2007. The Theory of Open Quantum Systems. Oxford University Press, New York.
- Brunelli, M., Olivares, S., & Paris, M. G. A. 2011. “Qubit thermometry for micromechanical resonators”. Phys. Rev. A, 84, 032105.
- Brunelli, M., Olivares, S., Paternostro, M., & Paris, M. G. A. 2012. “Qubit-assisted thermometry of a quantum harmonic oscillator”. Phys. Rev. A, 86, 012125.
- Campbell, S., Genoni, M. G., & Deffner, S. 2018a. “Precision thermometry and the quantum speed limit”. Quantum Sci. Technol., 3(2), 025002.
- Campbell, S., Genoni, M. G., & Deffner, S. 2018b. “Precision thermometry and the quantum speed limit”. Quantum Sci. Technol., 3(2), 025002.
- Cavina, V., Mancino, L., De Pasquale, A., Gianani, I., Sbroscia, M., Booth, R. I., Roccia, E., Raimondi, R., Giovannetti, V., & Barbieri, M. 2018. “Bridging thermodynamics and metrology in nonequilibrium quantum thermometry”. Phys. Rev. A, 98, 050101.
- Cenni, M. F., Lami, L., Acín, A., & Mehboudi, M. 2022. “Thermometry of Gaussian quantum systems using Gaussian measurements”. Quantum, 6, 743.
- Chowdhury, A., Vezio, P., Bonaldi, M., Borrielli, A., Marino, F., Morana, B., Pandraud, G., Pontin, A., Prodi, G. A., Sarro, P. M., Serra, E., & Marin, F. 2019a. “Calibrated quantum thermometry in cavity optomechanics”. Quantum Sci. Technol., 4(2), 024007.
- Chowdhury, A., Vezio, P., Bonaldi, M., Borrielli, A., Marino, F., Morana, B., Pandraud, G., Pontin, A., Prodi, G. A., Sarro, P. M., Serra, E., & Marin, F. 2019b. “Quantum Thermometry in Optomechanics”. In Quantum Information and Measurement (QIM) V: Quantum Technologies, (p. F5A.70). Optica Publishing Group.
- Chu, Y., Kharel, P., Renninger, W. H., Burkhart, L. D., Frunzio, L., Rakich, P. T., & Schoelkopf, R. J. 2017. “Quantum acoustics with superconducting qubits”. Science, 358(6360), 199–202.
- Correa, L. A., Mehboudi, M., Adesso, G., & Sanpera, A. 2015a. “Individual Quantum Probes for Optimal Thermometry”. Phys. Rev. Lett., 114, 220405.
- Correa, L. A., Mehboudi, M., Adesso, G., & Sanpera, A. 2015b. “Individual Quantum Probes for Optimal Thermometry”. Phys. Rev. Lett., 114, 220405.
- Correa, L. A., Perarnau-Llobet, M., Hovhannisyan, K. V., Hernández-Santana, S., Mehboudi, M., & Sanpera, A. 2017. “Enhancement of low-temperature thermometry by strong coupling”. Phys. Rev. A, 96, 062103.
- Cresser, J. 1992. “Thermal Equilibrium in the Jaynes-Cummings Model”. J. Mod. Opt., 39(11), 2187–2192.
- Damanet, F., & Martin, J. 2016. “Competition between finite-size effects and dipole–dipole interactions in few-atom systems”. J. Phys. B: At. Mol. Opt. Phys., 49(22), 225501.
- De Pasquale, A., & Stace, T. M. 2018. Quantum Thermometry. Cham: Springer International Publishing. Dedyulin, S., Ahmed, Z., & Machin, G. 2022. “Emerging technologies in the field of thermometry”. Meas. Sci. Technol., 33(9), 092001.
- Degen, C. L., Reinhard, F., & Cappellaro, P. 2017. “Quantum sensing”. Rev. Mod. Phys., 89, 035002. Diamanti, E., & Leverrier, A. 2015. “Distributing Secret Keys with Quantum Continuous Variables: Principle, Security and Implementations”. Entropy, 17(9), 6072–6092.
- Didier, N., Bourassa, J., & Blais, A. 2015. “Fast Quantum Nondemolition Readout by Parametric Modulation of Longitudinal Qubit-Oscillator Interaction”. Phys. Rev. Lett., 115, 203601.
- Dittmann, J. 1999. “Explicit formulae for the Bures metric”. J. Phys. A: Math. Gen., 32(14), 2663–2670. Eichenfield, M., Chan, J., Camacho, R. M., Vahala, K. J., & Painter, O. 2009. “Optomechanical crystals”. Nature, 462(7269), 78–82.
- Fang, K., Luo, J., Metelmann, A., Matheny, M. H., Marquardt, F., Clerk, A. A., & Painter, O. 2017. “Gen- eralized non-reciprocity in an optomechanical circuit via synthetic magnetism and reservoir engineering”. Nat. Phys., 13(5), 465–471.
- Feyles, M. M., Mancino, L., Sbroscia, M., Gianani, I., & Barbieri, M. 2019. “Dynamical role of quantum signatures in quantum thermometry”. Phys. Rev. A, 99, 062114.
- Gatti, A., Brambilla, E., & Lugiato, L. 2008. “Chapter 5 Quantum imaging”. vol. 51 of Prog. Opt., (pp. 251–348). Elsevier.
- Gelbwaser-Klimovsky, D., Alicki, R., & Kurizki, G. 2013. “Minimal universal quantum heat machine”. Phys. Rev. E, 87, 012140.
- Gelbwaser-Klimovsky, D., & Kurizki, G. 2014. “Heat-machine control by quantum-state preparation: From quantum engines to refrigerators”. Phys. Rev. E, 90, 022102.
- Gelbwaser-Klimovsky, D., & Kurizki, G. 2015. “Work extraction from heat-powered quantized optomechanical setups”. Sci. Rep., 5(1), 7809.
- Gianani, I., Farina, D., Barbieri, M., Cimini, V., Cavina, V., & Giovannetti, V. 2020. “Discrimination of thermal baths by single-qubit probes”. Phys. Rev. Research, 2, 033497.
- Giovannetti, V., Lloyd, S., & Maccone, L. 2001. “Quantum-enhanced positioning and clock synchronization”. Nature, 412(6845), 417–419.
- Giovannetti, V., Lloyd, S., & Maccone, L. 2011a. “Advances in quantum metrology”. Nat. Photon., 5(4), 222–229.
- Giovannetti, V., Lloyd, S., & Maccone, L. 2011b. “Advances in quantum metrology”. Nat. Photonics, 5(4), 222–229.
- Glatthard, J., & Correa, L. A. 2022. “Bending the rules of low-temperature thermometry with periodic driving”. Quantum, 6, 705.
- Glatthard, J., Rubio, J., Sawant, R., Hewitt, T., Barontini, G., & Correa, L. A. 2022. “Optimal Cold Atom Thermometry Using Adaptive Bayesian Strategies”. PRX Quantum, 3, 040330.
- Gupta, S., Moore, K. L., Murch, K. W., & Stamper-Kurn, D. M. 2007. “Cavity Nonlinear Optics at Low Photon Numbers from Collective Atomic Motion”. Phys. Rev. Lett., 99, 213601.
- He, B. 2012. “Quantum optomechanics beyond linearization”. Phys. Rev. A, 85, 063820. Helstrom, C. W. 1969. “Quantum detection and estimation theory”.
- Higgins, K. D. B., Lovett, B. W., & Gauger, E. M. 2013. “Quantum thermometry using the ac Stark shift within the Rabi model”. Phys. Rev. B, 88, 155409.
- Hong, T., Yang, H., Miao, H., & Chen, Y. 2013. “Open quantum dynamics of single-photon optomechanical devices”. Phys. Rev. A, 88, 023812.
- Hovhannisyan, K. V., & Correa, L. A. 2018. “Measuring the temperature of cold many-body quantum systems”. Phys. Rev. B, 98, 045101.
- Huntemann, N., Sanner, C., Lipphardt, B., Tamm, C., & Peik, E. 2016. “Single-Ion Atomic Clock with 3 × 10−18 Systematic Uncertainty”. Phys. Rev. Lett., 116, 063001.
- Çakmak, B. 2020. “Ergotropy from coherences in an open quantum system”. Phys. Rev. E, 102, 042111.
- Jevtic, S., Newman, D., Rudolph, T., & Stace, T. M. 2015. “Single-qubit thermometry”. Phys. Rev. A, 91, 012331.
- Jiang, Z. 2014. “Quantum Fisher information for states in exponential form”. Phys. Rev. A, 89, 032128.
- Johansson, J., Nation, P., & Nori, F. 2012. “QuTiP: An open-source Python framework for the dynamics of open quantum systems”. Comput. Phys. Commun., 183(8), 1760–1772.
- Johansson, J. R., Johansson, G., & Nori, F. 2014a. “Optomechanical-like coupling between superconducting resonators”. Phys. Rev. A, 90, 053833.
- Johansson, J. R., Johansson, G., & Nori, F. 2014b. “Optomechanical-like coupling between superconducting resonators”. Phys. Rev. A, 90, 053833.
- Jørgensen, M. R., Kołodyński, J., Mehboudi, M., Perarnau-Llobet, M., & Brask, J. B. 2022. “Bayesian quantum thermometry based on thermodynamic length”. Phys. Rev. A, 105, 042601.
- Jørgensen, M. R., Potts, P. P., Paris, M. G. A., & Brask, J. B. 2020. “Tight bound on finite-resolution quantum thermometry at low temperatures”. Phys. Rev. Research, 2, 033394.
- Kargı, C., Naseem, M. T., Opatrný, T. c., Müstecaplıoğlu, O. E., & Kurizki, G. 2019. “Quantum optical two-atom thermal diode”. Phys. Rev. E, 99, 042121.
- Khalili, F. Y., & Danilishin, S. L. 2016. “Chapter Three - Quantum Optomechanics”. vol. 61 of Progress in Optics, (pp. 113–236). Elsevier.
- Kiilerich, A. H., De Pasquale, A., & Giovannetti, V. 2018a. “Dynamical approach to ancilla-assisted quantum thermometry”. Phys. Rev. A, 98, 042124.
- Kiilerich, A. H., De Pasquale, A., & Giovannetti, V. 2018b. “Dynamical approach to ancilla-assisted quantum thermometry”. Phys. Rev. A, 98, 042124.
- Kosloff, R. 2013. “Quantum Thermodynamics: A Dynamical Viewpoint”. Entropy, 15(6), 2100–2128.
- Kronwald, A., Ludwig, M., & Marquardt, F. 2013. “Full photon statistics of a light beam transmitted through an optomechanical system”. Phys. Rev. A, 87, 013847.
- LaHaye, M. D., Suh, J., Echternach, P. M., Schwab, K. C., & Roukes, M. L. 2009. “Nanomechanical measurements of a superconducting qubit”. Nature, 459(7249), 960–964.
- Latune, C. L., Sinayskiy, I., & Petruccione, F. 2019. “Energetic and entropic effects of bath-induced coher- ences”. Phys. Rev. A, 99, 052105.
- Law, C. K. 1995. “Interaction between a moving mirror and radiation pressure: A Hamiltonian formulation”. Phys. Rev. A, 51, 2537–2541.
- Leanhardt, A. E., Pasquini, T. A., Saba, M., Schirotzek, A., Shin, Y., Kielpinski, D., Pritchard, D. E., & Ketterle, W. 2003. “Cooling Bose-Einstein Condensates Below 500 Picokelvin”. Science, 301(5639), 1513–1515.
- Leggett, A. J., Chakravarty, S., Dorsey, A. T., Fisher, M. P. A., Garg, A., & Zwerger, W. 1987. “Dynamics of the dissipative two-state system”. Rev. Mod. Phys., 59, 1–85.
- Lewenstein, M., Sanpera, A., Ahufinger, V., Damski, B., Sen(De), A., & Sen, U. 2007. “Ultracold atomic gases in optical lattices: mimicking condensed matter physics and beyond”. Advances in Physics, 56(2), 243–379.
- Liao, J.-Q., Cheung, H. K., & Law, C. K. 2012. “Spectrum of single-photon emission and scattering in cavity optomechanics”. Phys. Rev. A, 85, 025803.
- Lü, X.-Y., Zhang, W.-M., Ashhab, S., Wu, Y., & Nori, F. 2013. “Quantum-criticality-induced strong Kerr nonlinearities in optomechanical systems”. Sci. Rep., 3(1), 2943.
- Ludwig, M., Safavi-Naeini, A. H., Painter, O., & Marquardt, F. 2012. “Enhanced Quantum Nonlinearities in a Two-Mode Optomechanical System”. Phys. Rev. Lett., 109, 063601.
- Mancini, S., Man’ko, V. I., & Tombesi, P. 1997. “Ponderomotive control of quantum macroscopic coherence”. Phys. Rev. A, 55, 3042–3050.
- Mancino, L., Genoni, M. G., Barbieri, M., & Paternostro, M. 2020. “Nonequilibrium readiness and precision of Gaussian quantum thermometers”. Phys. Rev. Res., 2, 033498.
- Mancino, L., Sbroscia, M., Gianani, I., Roccia, E., & Barbieri, M. 2017. “Quantum Simulation of Single-Qubit Thermometry Using Linear Optics”. Phys. Rev. Lett., 118, 130502.
- Marian, P., & Marian, T. A. 2007. “Optimal purifications and fidelity for displaced thermal states”. Phys. Rev. A, 76, 054307.
- Marshall, W., Simon, C., Penrose, R., & Bouwmeester, D. 2003. “Towards Quantum Superpositions of a Mirror”. Phys. Rev. Lett., 91, 130401.
- Marti, G. E., Hutson, R. B., Goban, A., Campbell, S. L., Poli, N., & Ye, J. 2018. “Imaging Optical Frequencies with 100 μHz Precision and 1.1 μm Resolution”. Phys. Rev. Lett., 120, 103201.
- Massel, F., Cho, S. U., Pirkkalainen, J.-M., Hakonen, P. J., Heikkilä, T. T., & Sillanpää, M. A. 2012. “Multimode circuit optomechanics near the quantum limit”. Nat. Commun., 3(1), 987.
- Massel, F., Heikkilä, T. T., Pirkkalainen, J.-M., Cho, S. U., Saloniemi, H., Hakonen, P. J., & Sillanpää, M. A. 2011. “Microwave amplification with nanomechanical resonators”. Nature, 480(7377), 351–354.
- Medley, P., Weld, D. M., Miyake, H., Pritchard, D. E., & Ketterle, W. 2011. “Spin Gradient Demagnetization Cooling of Ultracold Atoms”. Phys. Rev. Lett., 106, 195301.
- Mehboudi, M., Jørgensen, M. R., Seah, S., Brask, J. B., Kołodyński, J., & Perarnau-Llobet, M. 2022. “Fundamental Limits in Bayesian Thermometry and Attainability via Adaptive Strategies”. Phys. Rev. Lett., 128, 130502.
- Mehboudi, M., Moreno-Cardoner, M., Chiara, G. D., & Sanpera, A. 2015. “Thermometry precision in strongly correlated ultracold lattice gases”. New J. Phys., 17(5), 055020.
- Mehboudi, M., Sanpera, A., & Correa, L. A. 2019. “Thermometry in the quantum regime: Recent theoretical progress”. J. Phys. A: Math. Theor., 52(30), 303001.
- Mirkhalaf, S. S., Mehboudi, M., Qaleh, Z. N., & Rahimi-Keshari, S. 2023. “Operational significance of nonclassicality in nonequilibrium Gaussian quantum thermometry”.
- Mok, W.-K., Bharti, K., Kwek, L.-C., & Bayat, A. 2021. “Optimal probes for global quantum thermometry”. Commun. Phys., 4(1), 62.
- Montenegro, V., Genoni, M. G., Bayat, A., & Paris, M. G. A. 2020. “Mechanical oscillator thermometry in the nonlinear optomechanical regime”. Phys. Rev. Res., 2, 043338.
- Montenegro, V., Genoni, M. G., Bayat, A., & Paris, M. G. A. 2022. “Probing of nonlinear hybrid optome- chanical systems via partial accessibility”. Phys. Rev. Res., 4, 033036.
- Mukherjee, V., Zwick, A., Ghosh, A., Chen, X., & Kurizki, G. 2019. “Enhanced precision bound of low- temperature quantum thermometry via dynamical control”. Commun. Phys., 2(1), 162.
- Naseem, M. T., Misra, A., & Müstecaplioğlu, O. E. 2020a. “Two-body quantum absorption refrigerators with optomechanical-like interactions”. Quantum Sci. Technol., 5(3), 035006.
- Naseem, M. T., Misra, A., Müstecaplioğlu, O. E., & Kurizki, G. 2020b. “Minimal quantum heat manager boosted by bath spectral filtering”. Phys. Rev. Research, 2, 033285.
- Naseem, M. T., & Müstecaplıoğlu, O. E. 2022. “Antibunching via cooling by heating”. Phys. Rev. A, 105, 012201.
- Naseem, M. T., Xuereb, A., & Müstecaplıoğlu, O. E. 2018. “Thermodynamic consistency of the optomechan- ical master equation”. Phys. Rev. A, 98, 052123.
- Navascués, M., & Acín, A. 2005. “SecurityBounds for Continuous Variables Quantum Key Distribution”. Phys. Rev. Lett., 94, 020505.
- Neumann, e. a., P. 2013. “High-Precision Nanoscale Temperature Sensing Using Single Defects in Diamond”. Nano Letters, 13(6), 2738–2742.
- Nichols, R., Liuzzo-Scorpo, P., Knott, P. A., & Adesso, G. 2018. “Multiparameter Gaussian quantum metrology”. Phys. Rev. A, 98, 012114.
- Oz-Vogt, J., Mann, A., & Revzen, M. 1991. “Thermal Coherent States and Thermal Squeezed States”. J. Mod. Opt., 38(12), 2339–2347.
- Paris, M. G. A. 2009. “Quantum estimation for quantum technology”. Intl. J. Quant. Inf., 07(supp01), 125–137.
- Petrosyan, D., Bensky, G., Kurizki, G., Mazets, I., Majer, J., & Schmiedmayer, J. 2009. “Reversible state transfer between superconducting qubits and atomic ensembles”. Phys. Rev. A, 79, 040304.
- Pinel, O., Jian, P., Treps, N., Fabre, C., & Braun, D. 2013. “Quantum parameter estimation using general single-mode Gaussian states”. Phys. Rev. A, 88, 040102.
- Pirkkalainen, J.-M., Cho, S. U., Massel, F., Tuorila, J., Heikkilä, T. T., Hakonen, P. J., & Sillanpää, M. A. 2015. “Cavity optomechanics mediated by a quantum two-level system”. Nat. Commun., 6(1), 6981.
- Planella, G., Cenni, M. F. B., Acín, A., & Mehboudi, M. 2022. “Bath-Induced Correlations Enhance Ther- mometry Precision at Low Temperatures”. Phys. Rev. Lett., 128, 040502.
- Purdy, T. P., Grutter, K. E., Srinivasan, K., & Taylor, J. M. 2017. “Quantum correlations from a room- temperature optomechanical cavity”. Science, 356(6344), 1265–1268.
- Qvarfort, S., Plato, A. D. K., Bruschi, D. E., Schneiter, F., Braun, D., Serafini, A., & Rätzel, D. 2021. “Optimal estimation of time-dependent gravitational fields with quantum optomechanical systems”. Phys. Rev. Res., 3, 013159.
- Qvarfort, S., Serafini, A., Barker, P. F., & Bose, S. 2018. “Gravimetry through non-linear optomechanics”. Nature Communications, 9(1), 3690.
- Rabl, P. 2011. “Photon Blockade Effect in Optomechanical Systems”. Phys. Rev. Lett., 107, 063601.
- Restrepo, J., Ciuti, C., & Favero, I. 2014. “Single-Polariton Optomechanics”. Phys. Rev. Lett., 112, 013601.
- Richer, S., & DiVincenzo, D. 2016. “Circuit design implementing longitudinal coupling: A scalable scheme for superconducting qubits”. Phys. Rev. B, 93, 134501.
- Rouxinol, F., Hao, Y., Brito, F., Caldeira, A. O., Irish, E. K., & LaHaye, M. D. 2016. “Measurements of nanoresonator-qubit interactions in a hybrid quantum electromechanical system”. Nanotechnology, 27(36), 364003.
- Rubio, J., Anders, J., & Correa, L. A. 2021. “Global Quantum Thermometry”. Phys. Rev. Lett., 127, 190402. Safavi-Naeini, A. H., Chan, J., Hill, J. T., Gröblacher, S., Miao, H., Chen, Y., Aspelmeyer, M., & Painter, O. 2013. “Laser noise in cavity-optomechanical cooling and thermometry”. New J. Phys., 15(3), 035007.
- Sala, K., Doicin, T., Armour, A. D., & Tufarelli, T. 2021. “Quantum estimation of coupling strengths in driven-dissipative optomechanics”. Phys. Rev. A, 104, 033508.
- Santos, J. P., Céleri, L. C., Landi, G. T., & Paternostro, M. 2019. “The role of quantum coherence in non-equilibrium entropy production”. npj Quantum Inf., 5(1), 23.
- Schneiter, F., Qvarfort, S., Serafini, A., Xuereb, A., Braun, D., Rätzel, D., & Bruschi, D. E. 2020. “Optimal estimation with quantum optomechanical systems in the nonlinear regime”. Phys. Rev. A, 101, 033834.
- Sekatski, P., & Perarnau-Llobet, M. 2022. “Optimal nonequilibrium thermometry in Markovian environ- ments”. Quantum, 6, 869.
- Sletten, L. R., Moores, B. A., Viennot, J. J., & Lehnert, K. W. 2019. “Resolving Phonon Fock States in a Multimode Cavity with a Double-Slit Qubit”. Phys. Rev. X, 9, 021056.
- Souza, M. d., Paupitz, R., Seridonio, A., & Lagos, R. E. 2016. “Specific Heat Anomalies in Solids Described by a Multilevel Model”. Braz. J. Phys., 46(2), 206–212.
- Spengler, F., Rätzel, D., & Braun, D. 2022. “Perspectives of measuring gravitational effects of laser light and particle beams”. New J. Phys., 24(5), 053021.
- Stannigel, K., Komar, P., Habraken, S. J. M., Bennett, S. D., Lukin, M. D., Zoller, P., & Rabl, P. 2012. “Optomechanical Quantum Information Processing with Photons and Phonons”. Phys. Rev. Lett., 109, 013603.
- Taylor, J. M., Cappellaro, P., Childress, L., Jiang, L., Budker, D., Hemmer, P. R., Yacoby, A., Walsworth, R., & Lukin, M. D. 2008. “High-sensitivity diamond magnetometer with nanoscale resolution”. Nat. Phys., 4(10), 810–816.
- Taylor, M. A., Janousek, J., Daria, V., Knittel, J., Hage, B., Bachor, H.-A., & Bowen, W. P. 2013. “Biological measurement beyond the quantum limit”. Nature Photonics, 7(3), 229–233.
- Ullah, A., Naseem, M. T., & Müstecaplıoğlu, O. E. 2023a. “Low-temperature quantum thermometry boosted by coherence generation”. Phys. Rev. Res., 5, 043184.
- Ullah, A., Naseem, M. T., & Özgür E. Müstecaplıoğlu 2023b. “Preparation of thermal coherent state and its role in quantum thermometry”.
- Ullah, A., Pedram, A., Naseem, M. T., & Özgür E. Müstecaplıoğlu 2023c. “Quantum thermometry with an optomechanical system”.
- Vogel, W., & Welsch, D.-G. 2006. Quantum optics. John Wiley & Sons.
- Wang, X., Miranowicz, A., Li, H.-R., & Nori, F. 2016. “Method for observing robust and tunable phonon blockade in a nanomechanical resonator coupled to a charge qubit”. Phys. Rev. A, 93, 063861.
- Weedbrook, C., Pirandola, S., García-Patrón, R., Cerf, N. J., Ralph, T. C., Shapiro, J. H., & Lloyd, S. 2012. “Gaussian quantum information”. Rev. Mod. Phys., 84, 621–669.
- Wollack, E. A., Cleland, A. Y., Gruenke, R. G., Wang, Z., Arrangoiz-Arriola, P., & Safavi-Naeini, A. H. 2022. “Quantum state preparation and tomography of entangled mechanical resonators”. Nature, 604(7906), 463–467.
- Xiang, Z.-L., Ashhab, S., You, J. Q., & Nori, F. 2013. “Hybrid quantum circuits: Superconducting circuits interacting with other quantum systems”. Rev. Mod. Phys., 85, 623–653.
- Xie, D., Sun, F.-X., & Xu, C. 2020. “Quantum thermometry based on a cavity-QED setup”. Phys. Rev. A, 101, 063844.
- Xu, X.-W., Li, Y.-J., & Liu, Y.-x. 2013. “Photon-induced tunneling in optomechanical systems”. Phys. Rev. A, 87, 025803.
- Zhang, W.-M., Feng, D. H., & Gilmore, R. 1990. “Coherent states: Theory and some applications”. Rev. Mod. Phys., 62, 867–927.
- Zhong, W., Sun, Z., Ma, J., Wang, X., & Nori, F. 2013. “Fisher information under decoherence in Bloch representation”. Phys. Rev. A, 87, 022337.
- Zhou, X., Cattiaux, D., Gazizulin, R. R., Luck, A., Maillet, O., Crozes, T., Motte, J.-F., Bourgeois, O., Fefferman, A., & Collin, E. 2019. “On-chip Thermometry for Microwave Optomechanics Implemented in a Nuclear Demagnetization Cryostat”. Phys. Rev. Appl., 12, 044066.
APA | mustecaplioglu o, ULLAH A (2023). Etkileşen Sistemler ile Kuantum Termometreler. , 0 - 64. 122F371 |
Chicago | mustecaplioglu ozgur,ULLAH ASGHAR Etkileşen Sistemler ile Kuantum Termometreler. (2023): 0 - 64. 122F371 |
MLA | mustecaplioglu ozgur,ULLAH ASGHAR Etkileşen Sistemler ile Kuantum Termometreler. , 2023, ss.0 - 64. 122F371 |
AMA | mustecaplioglu o,ULLAH A Etkileşen Sistemler ile Kuantum Termometreler. . 2023; 0 - 64. 122F371 |
Vancouver | mustecaplioglu o,ULLAH A Etkileşen Sistemler ile Kuantum Termometreler. . 2023; 0 - 64. 122F371 |
IEEE | mustecaplioglu o,ULLAH A "Etkileşen Sistemler ile Kuantum Termometreler." , ss.0 - 64, 2023. 122F371 |
ISNAD | mustecaplioglu, ozgur - ULLAH, ASGHAR. "Etkileşen Sistemler ile Kuantum Termometreler". (2023), 0-64. https://doi.org/122F371 |
APA | mustecaplioglu o, ULLAH A (2023). Etkileşen Sistemler ile Kuantum Termometreler. , 0 - 64. 122F371 |
Chicago | mustecaplioglu ozgur,ULLAH ASGHAR Etkileşen Sistemler ile Kuantum Termometreler. (2023): 0 - 64. 122F371 |
MLA | mustecaplioglu ozgur,ULLAH ASGHAR Etkileşen Sistemler ile Kuantum Termometreler. , 2023, ss.0 - 64. 122F371 |
AMA | mustecaplioglu o,ULLAH A Etkileşen Sistemler ile Kuantum Termometreler. . 2023; 0 - 64. 122F371 |
Vancouver | mustecaplioglu o,ULLAH A Etkileşen Sistemler ile Kuantum Termometreler. . 2023; 0 - 64. 122F371 |
IEEE | mustecaplioglu o,ULLAH A "Etkileşen Sistemler ile Kuantum Termometreler." , ss.0 - 64, 2023. 122F371 |
ISNAD | mustecaplioglu, ozgur - ULLAH, ASGHAR. "Etkileşen Sistemler ile Kuantum Termometreler". (2023), 0-64. https://doi.org/122F371 |