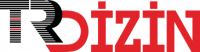
A Review of Biomedical Engineering Research in Turkey During the Period 2008-2018
Yıl: 2019 Cilt: 31 Sayı: 4 Sayfa Aralığı: 316 - 327 Metin Dili: İngilizce DOI: 10.7240/jeps.578024 İndeks Tarihi: 09-04-2020
A Review of Biomedical Engineering Research in Turkey During the Period 2008-2018
Öz: Biomedical engineering is one of the fastest developing research disciplines in the past 60 years with the aid of rapid advances in technology.Biomedical engineering has emerged in Turkey in late 1970s but the research conducted in this area has been developing only in thepast 15 years. The aim of this review is to summarize the problems regarding biomedical engineering in Turkey; to present the main subjectsthat are conducted in biomedical field in Turkey; and to summarize the prominent research papers conducted by Turkish Institutespublished during the period 2008-2018 that contribute and/or have a potential to contribute to research and development (R&D) in biomedicalengineering field in Turkey. These studies were divided into categories of tissue engineering, biosensors and biomedical devices;and summarized in this review.
Anahtar Kelime: Türkiye’de 2008-2018 Döneminde Yapılan Biyomedikal Mühendisliğindeki Araştırmaların Derlemesi
Öz: Biyomedikal mühendisliği, teknolojideki hızlı gelişmelerin yardımıyla son 60 yılda en hızlı gelişen araştırma disiplinlerinden biri olmuştur. Biyomedikal mühendisliği Türkiye’de 1970’lerin sonunda ortaya çıkmış, ancak bu alanda yapılan araştırmalar sadece son 15 yılda gelişmeye başlamıştır. Bu derlemenin amacı, Türkiye’de biyomedikal mühendisliği ile ilgili sorunları özetlemek; Türkiye’de biyomedikal alanında yürütülen ana konuları sunmak; ve 2008-2018 yılları arasında yayınlanan ve Türkiye’de biyomedikal mühendisliği alanında araştırma ve geliştirmeye (AR-GE) katkıda bulunan/bulunma potansiyeline sahip olan ve Türk Enstitüleri tarafından yürütülen önde gelen araştırma makalelerini özetlemektir. Bu araştırmalar doku mühendisliği, biyosensörler ve biyomedikal cihazlar konularına ayrılarak incelenmiştir.
Anahtar Kelime: Belge Türü: Makale Makale Türü: Araştırma Makalesi Erişim Türü: Erişime Açık
- [1] Bronzino, J.D., (2012). Biomedical Engineering: A Historical Perspective. In Introduction to Biomedical Engineering, J.D. Bronzino, J.D. Enderle (ed.), 1-33, doi: 10.1016/B978-0-12- 374979-6.00001-0.
- [2] Saltzman, W.M., (2015). Biomedical engineering: bridging medicine and technology, Second Edi. Cambridge University Press.
- [3] Nebeker, F., (2002). Golden accomplishments in biomedical engineering. IEEE Eng. Med. Biol. Mag., 21:17–47.
- [4] Humpolíčk, P., Radaszkiewicz, K.A., Capáková, Z., Pacherník, J., Bober, P., Kašpárková, V., Rejmontová, P., Lehocký, M., Ponížil, P., Stejskal, J., (2018). Polyaniline cryogels: Biocompatibility of novel conducting macroporous material. Sci. Rep., 8:1–12.
- [5] Requena-Carrion, J., & Leder, R. S. (2009, September). The natural history of the engineering in medicine and biology ociety from a modern perspective. In 2009 Annual International Conference of the IEEE Engineering in Medicine and Biology Society (pp. 1086-1088). IEEE.
- [6] Schwan, H.P., (1991). Biomedical engineering: University of Pennsylvania-from research laboratory to a leader in educational institutions for bioengineering. IEEE Eng Med. Biol. Mag., 10:47–49.
- [7] Sun, H.H., (1991). Biomedical engineering: Drexel University- pioneer in a formal MS degree training program for doctors. IEEE Eng. Med. Biol. Mag., 10:44–46.
- [8] Çamurcu, A.Y., Alsan, S., (1998). Türkiye’de ve Dünyada Biyomedikal Mühendislik ve Biyomedikal Cihaz Teknolojisi Eğitimi. Atatürk Eğitim Fakültesi Eğitim Bilimleri Dergisi, 10: 51–58.
- [9] Sezdi, M., Akan, A., Kalkandelen, C., (2009). Biyomedikal ve Klinik Mühendisliği Eğitimi ve Ülkemizin Bu Alandaki İhtiyaçlarının İncelenmesi. EEBB09 Elektrik Elektronik Bilgisayar Biyomedikal Mühendislikleri Eğitimi 4. Ulus. Sempozyumu. Eskişehir, Türkiye, 22-24.
- [10] Yükseköğretim Kurulu Yükseköğretim Program Atlası. https:// yokatlas.yok.gov.tr/#. Accessed 29 Apr 2018.
- [11] World University Rankings & Reviews uniRank. https:// www.4icu.org/tr/. Accessed 20 May 2019.
- [12] European Research Area Progress Report 2016. https:// ec.europa.eu/research/era/pdf/era_progress_report2016/era_ progress_report_2016_com.pdf. Accessed 20 March 2018.
- [13] Taşgetiren, S., Yuran, F., Özmen, N., Özkan, N., (2015). Biyomedikal Ar-Ge 2015: 2015 İtibariyle Türkiye’de Biyomedikal Teknolojileri Alanında Yapılan Araştırma Faaliyetlerinin Mevcut Durumu. doi: 10.13140/2.1.3053.9043.
- [14] Bozer, A., Ağırbaş, İ., (2016). Tıbbi Görüntüleme Cihazlarının Sayısal Durumu ve Kullanımlarının Değerlendirilmesi – Quantitative Evaluation of the Status and Use of Medical Imaging Devices. Ankara Üniversitesi Tıp Fakültesi Macmuası, 69(3), 193-201, doi: DOI: 10.1501/Tıpfak_000000943.
- [15] Kiper, M., (2013). Türkiye’de tıbbi cihaz sektörü ve strateji önerisi. Türkiye Teknol. Geliştirme Vakfı, 1–226.
- [16] Mertler, A.A., Karadoğan, N., Tatarhan, G., (2015). Türkiye’de Tıbbi Cihazların Sayısal Durumu ve OECD Ülkeleri İle Karşılaştırmaları. Int. J. Heal. Manag. Strateg. Res. 1:52–70.
- [17] T.C. Sağlık Bakanlığı, (2013). Sağlık İstatistikleri Yıllığı 2013. Sentez Matbaacılık ve Yayıncılık, Ankara.
- [18] Türkiye Istatistik Kurumu Türkiye İstatistik Kurumu. http:// www.tuik.gov.tr/PreTablo.do?alt_id=1084. Accessed 20 March 2018.
- [19] Ministry of Health of the Republic of Turkey. Health Treansformation Programme.https://sbu.saglik.gov.tr/Ekutuphane/ kitaplar/healthtransformation.pdf. Accessed 20 March 2018.
- [20] Bakker, E., Nuijens, R., Kaplan, D., (2015). The Turkish life sciences and health sector: Identifying opportunities to exchange knowledge and products between the Netherlands and Turkey. https://healthholland.h5mag.com/healthholland/ edit-update_may_2015/internationalisation/1944/ Report_Turkish_Life_Sciences_and_Health_Sector_ March_2015__1_.pdf?nocache=113.117.7427. Accessed 20 July 2018.
- [21] Dundar, M., Akbarova, Y., (2011). Current State of Biotechnology in Turkey. Curr. Opin. Biotechnol., 22S:S3-S6 doi: 10.1016/j.copbio.2011.05.509.
- [22] Eurostat Research Projects under Framework Programmes – European Commission. https://ec.europa.eu/eurostat/cros/ content/research-projects-under-framework-programmes-0_ en. Accessed 1 May 2018.
- [23] European Commission – PRESS RELEASES – Press release – Turkey joins Horizon 2020 research and innovation programme. http://europa.eu/rapid/press-release_IP-14-631_ en.htm. Accessed 1 May 2018.
- [24] Yaşar, T., (2017). TÜBİTAK Türkiye Adresli Uluslararası Bilimsel Yayınları Teşvik (UBYT) Programının Değerlendirilmes. TÜBİTAK Ulakbim.
- [25] SciVerse SCOPUS. www.scopus.com. Accessed 22 January 2019.
- [26] Health European Commission. https://ec.europa.eu/programmes/ horizon2020/en/area/ health. Accessed 1 May 2018.
- [27] Morouço, P., Biscaia, S., Viana, T., Franco, M., Malça, C., Mateus, A., Moura, C., Ferreira, F.C., Mitchell, G., Alves, N.M., (2016). Fabrication of poly(ϵ-caprolactone) scaffolds reinforced with cellulose nanofibers, with and without the addition of hydroxyapatite nanoparticles. Biomed. Res. Int., 1-10, doi: 10.1155/2016/1596157.
- [28] Andreu, V., Mendoza, G., Arruebo, M., Irusta, S., (2015). Smart dressings based on nanostructured fibers containing natural origin antimicrobial, anti-inflammatory, and regenerative compounds. Materials (Basel), 8:5154–5193.
- [29] O’Brien, F.J., (2011). Biomaterials & scaffolds for tissue engineering. Mater. Today, 14:88–95.
- [30] Langer, R., Tirrell, D.A., (2004). Designing materials for biology and medicine. Nature, 428:487–492.
- [31] Kulkarni, R.K., Pani, K.C., Neuman, C., Leonard, F., (1966). Polylactic acid for surgical implants. Arch. Surg., 93:839– 843.
- [32] Vert, M., Mauduit, J., Li, S., (1994). Biodegradation of PLA/ GA polymers: increasing complexity. Biomaterials, 15:1209– 13.
- [33] Weir, N.A., Buchanan, F.J., Orr, J.F., Farrar, D.F., Boyd, A., (2004). Processing, annealing and sterilisation of poly-L-lactide. Biomaterials, 25:3939–3949.
- [34] Zaman, H.U., Islam, J.M.M., Khan, M.A., Khan, R.A., (2011). Physico-mechanical properties of wound dressing material and its biomedical application. J. Mech. Behav. Biomed. Mater., 4:1369–1375.
- [35] Zorlutuna, P., Yilgör P., Başmanav, F.B., Hasirci, V., (2009). Biomaterials and tissue engineering research in Turkey: The METU biomat center experience. Biotechnol. J., 4:965–980.
- [36] Karahaliloǧlu, Z., Demirbilek, M., Şam, M., Erol-Demirbilek, M., Saǧlam, N., Denkbaş, E.B., (2013). Plasma polymerization- modified bacterial polyhydroxybutyrate nanofibrillar scaffolds. J. Appl. Polym. Sci., 128:1904–1912.
- [37] Şen, Ö., Culha, M., (2016). Boron nitride nanotubes included thermally cross-linked gelatin-glucose scaffolds show improved properties. Colloids Surfaces B. Biointerfaces 138:41– 49.
- [38] Bhullar, S.K., Rana, D., Lekesiz, H., Bedeloglu, A.C., Ko, J., Cho, Y., Aytac, Z., Uyar, T., Jun, M., Ramalingam, M., (2017). Design and fabrication of auxetic PCL nanofiber membranes for biomedical applications. Mater. Sci. Eng. C, 81:334–340.
- [39] Yilgor, P., Tuzlakoglu, K., Reis, R.L., Hasirci, N., Hasirci, V., (2009). Incorporation of a sequential BMP-2/BMP-7 delivery system into chitosan-based scaffolds for bone tissue engineering. Biomaterials, 30:3551–3559.
- [40] Basmanav, F.B., Kose, G.T., Hasirci, V., (2008). Sequential growth factor delivery from complexed microspheres for bone tissue engineering. Biomaterials, 29:4195–4204.
- [41] Mavis, B., Demirtaş, T.T., Gümüşderelioğlu, M., Gündüz, G., Çolak, Ü. (2009). Synthesis, characterization and osteoblastic activity of polycaprolactone nanofibers coated with biomimetic calcium phosphate. Acta Biomater., 5:3098–3111.
- [42] Erol, M., Özyuĝuran, A., Özarpat, Ö., Küçükbayrak, S., (2012). 3D Composite scaffolds using strontium containing bioactive glasses. J. Eur. Ceram. Soc., 32:2747–2755.
- [43] Gunduz, O., Gode, C., Ahmad, Z., Gökçe, H., Yetmez, M., Kalkandelen, C., Sahin, Y.M., Oktar, F.N., (2014). Preparation and evaluation of cerium oxide-bovine hydroxyapatite composites for biomedical engineering applications. J. Mech. Behav. Biomed. Mater., 35:70–76.
- [44] Saber-Samandari, S., Alamara, K., Saber-Samandari, S., Gross, K.A., (2013). Micro-Raman spectroscopy shows how the coating process affects the characteristics of hydroxylapatite. Acta Biomater., 9:9538–9546.
- [45] Deliormanli, A.M., (2014). Preparation and in vitro characterization of electrospun 45S5 bioactive glass nanofibers. Ceram. Int., 41:417–425.
- [46] Doǧan, A., Yalvaç, M.E., Şahin, F., Kabanov, A. V., Palotás, A., Rizvanov, A.A., (2012). Differentiation of human stem cells is promoted by amphiphilic pluronic block copolymers. Int. J. Nanomedicine, 7:4849–4860.
- [47] Akin, H., Tugut F., Akin, G.E., Guney, U., Mutaf, B., (2012). Effect of Er:YAG laser application on the shear bond strength and microleakage between resin cements and Y-TZP ceramics. Lasers Med. Sci., 27:333–338.
- [48] Karahaliloglu, Z., Ercan, B., Taylor, E.N., Chung, S., Denkbaş, E.B., Webster, T.J., (2015). Antibacterial Nanostructured Polyhydroxybutyrate Membranes for Guided Bone Regeneration. J. Biomed. Nanotechnol., 11:2253–2263.
- [49] Kaya, M., Baran, T., Mentes, A., Asaroglu, M., Sezen, G., Tozak, K.O., (2014). Extraction and Characterization of α-Chitin and Chitosan from Six Different Aquatic Invertebrates. Food Biophys., 9:145–157.
- [50] Sahiner, N., Sagbas, S., Aktas, N., (2015). Single step natural poly(tannic acid) particle preparation as multitalented biomaterial. Mater. Sci. Eng. C, 49:824–834.
- [51] Işiklan, N., Küçükbalci, G., (2012). Microwave-induced synthesis of alginate-graft-poly(N-isopropylacrylamide) and drug release properties of dual pH – and temperature-responsive beads. Eur. J. Pharm. Biopharm., 82:316–331.
- [52] Yucel, D., Kose, G.T., Hasirci, V., (2010). Polyester based nerve guidance conduit design. Biomaterials, 31:1596–1603.
- [53] Irimia Vladu, M., Troshin, P.A., Reisinger, M., Shmygleva, L., Kanbur, Y., Schwabegger, G., Bodea, M., Schwödiauer, R., Mumyatov, A., Fergus, J.W., (2010). Biocompatible and biodegradable materials for organic field effect transistors. Adv. Funct. Mater., 20:4069–4076.
- [54] Algi, M.P., Okay, O., (2014). Highly stretchable self-healing poly(N,N-dimethylacrylamide) hydrogels. Eur. Polym. J., 59:113–121.
- [55] Akturk, O., Tezcaner, A., Bilgili, H., Deveci, M.S., Gecit, M.R., Keskin, D. (2011). Evaluation of sericin/collagen membranes as prospective wound dressing biomaterial. J. Biosci. Bioeng., 112:279–288.
- [56] Uysal, C.A., Tobita, M., Hyakusoku, H., Mizuno, H., (2012). Adipose-derived stem cells enhance primary tendon repair: biomechanical and immunohistochemical evaluation. J. Plast. Reconstr. Aesthetic Surg., 65:1712–1719.
- [57] Wang, W., Xu, Y., Li, A., Li, T., Liu, M., von Klitzing, R., Ober, C.K., Kayitmazer, A..B, Li, L., Guo, X., (2015). Zinc induced polyelectrolyte coacervate bioadhesive and its transition to a self-healing hydrogel. Rsc. Adv., 5:66871–66878.
- [58] Eke, G., Mangir, N., Hasirci, N., MacNeil, S., Hasirci, V., (2017). Development of a UV crosslinked biodegradable hydrogel containing adipose derived stem cells to promote vascularization for skin wounds and tissue engineering. Biomaterials, 129:188–198.
- [59] Mammadov, R., Mammadov, B., Toksoz, S., Aydin,. B, Yagci, R., Tekinay, A.B., Guler, M.O., (2011). Heparin mimetic peptide nanofibers promote angiogenesis. Biomacromolecules, 12:3508–3519.
- [60] Uzunalli, G., Mammadov, R., Yesildal, F., Alhan, D., Ozturk, S., Ozgurtas, T., Guler, M.O., Tekinay, A.B., (2016). Angiogenic heparin-mimetic peptide nanofiber gel improves regenerative healing of acute wounds. ACS Biomater. Sci. Eng., 3:1296–1303.
- [61] Umut, E., Pineider, F., Arosio, P., Sangregorio, C., Corti, M., Tabak, F., Lascialfari, A., Ghigna, P., (2012). Magnetic, optical and relaxometric properties of organically coated gold-magnetite (Au-Fe3O4) hybrid nanoparticles for potential use in biomedical applications. J. Magn. Magn. Mater., 324:2373–2379.
- [62] Bürck, J., Aras, O., Bertinetti, L., Ilhan, C.A., Ermeydan, M.A., Schneider, R., Ulrich, A.S., Kazanci, M., (2018). Observation of triple helix motif on electrospun collagen nanofibers and its effect on the physical and structural properties. J. Mol. Struct., 1151:73–80.
- [63] Aras, O., Kazanci, M., (2015). Production of collagen micro- and nanofibers for potential drug-carrier systems. J. Enzyme Inhib. Med. Chem., 30:1013–1016.
- [64] Kazanci, M., (2014). Solvent and temperature effects on folding of electrospun collagen nanofibers. Mater. Lett., 130:223–226.
- [65] Unsoy, G., Yalcin, S., Khodadust, R., Gunduz, G., Gunduz, U., (2012). Synthesis optimization and characterization of chitosan-coated iron oxide nanoparticles produced for biomedical applications. J. Nanoparticle Res., 14:964.
- [66] Xu, F., Inci, F., Mullick, O., Atakan, U., Sung, Y., Kavaz, D., (2012). Release of Magnetic Nanoparticles from Cell – Encapsulating Biodegradable Nanobiomaterials. ACS nano, 2012, 6.8: 6640-6649.
- [67] Erdal, E., Kavaz, D., Şam, M., Demirbilek, M., Demirbilek, M.E., Saǧlam, N., Denkbaş, E.B., (2012). Preparation and characterization of magnetically responsive bacterial polyester based nanospheres for cancer therapy. J. Biomed. Nanotechnol., 8:800–808.
- [68] Nazli, C., Ergenc, T.I., Yar, Y., Acar, H.Y., Kizilel, S., (2012). RGDS-functionalized polyethylene glycol hydrogel-coated magnetic iron oxide nanoparticles enhance specific intracellular uptake by HeLa cells. Int. J. Nanomedicine, 7:1903– 1920.
- [69] Tamer, U., Gündoǧdu, Y., Boyaci, I.H., Pekmez, K., (2010). Synthesis of magnetic core-shell Fe3O4-Au nanoparticle for biomolecule immobilization and detection. J Nanoparticle Res., 12:1187–1196.
- [70] Silan, C., Akcali, A., Otkun, M.T., Ozbey, N., Butun, S., Ozay, O., Sahiner, N., (2012). Novel hydrogel particles and their IPN films as drug delivery systems with antibacterial properties. Colloids Surfaces B. Biointerfaces, 89:248–253.
- [71] Ercan, B., Taylor, E., Alpaslan, E., Webster, T.J., (2011). Diameter of titanium nanotubes influences anti-bacterial efficacy. Nanotechnology, 22:295102.
- [72] Demirci, S., Ustaoǧlu, Z., Yilmazer, G.A., Sahin, F., Baç, N., (2014). Antimicrobial properties of zeolite-X and zeolite- A ion-exchanged with silver, copper, and zinc against a broad range of microorganisms. Appl. Biochem. Biotechnol., 172:1652–1662.
- [73] Khandpur, R.S., (2003). Handbook of biomedical instrumentation, Second Edi. Tata McGraw-Hill Education.
- [74] Turner, A.P.F., (2013). Biosensors: sense and sensibility. Chem. Soc. Rev., 42:3184–3196.
- [75] Alwarappan, S., Erdem, A., Liu, C., Li, C-Z., (2009). Probing the electrochemical properties of graphene nanosheets for biosensing applications. J. Phys. Chem. C., 113:8853–8857.
- [76] Iverson, N.M., Barone, P.W., Shandell, M., Trudel, L.J., Sen, S., Sen, F., Ivanov, V., Atolia, E., Farias, E., McNicholas, T.P., (2013). In vivo biosensing via tissue-localizable near-infrared- fluorescent single-walled carbon nanotubes. Nat. Nanotechnol., 8:873.
- [77] Kaçar, C., Dalkiran, B., Erden, P.E., Kiliç, E., (2014). An amperometric hydrogen peroxide biosensor based on Co3O4 nanoparticles and multiwalled carbon nanotube modified glassy carbon electrode. Appl. Surf. Sci., 311:139–146.
- [78] Canbay, E., Şahin, B., Kiran, M., Akyilmaz, E., (2015). MWCNT- cysteamine-Nafion modified gold electrode based on myoglobin for determination of hydrogen peroxide and nitrite. Bioelectrochemistry, 101:126–131.
- [79] Korkut, S., Keskinler, B., Erhan, E., (2008). An amperometric biosensor based on multiwalled carbon nanotube-poly (pyrrole)- horseradish peroxidase nanobiocomposite film for determination of phenol derivatives. Talanta, 76:1147–1152.
- [80] Çevik, E., Şenel, M., Baykal, A., Abasiyanik, M.F., (2012). A novel amperometric phenol biosensor based on immobilized HRP on poly(glycidylmethacrylate)-grafted iron oxide nanoparticles for the determination of phenol derivatives. Sensors Actuators, B. Chem., 173:396–405.
- [81] Dalkiran, B., Kaçar, C., Erden, P.E., Kiliç, E., (2014). Amperometric xanthine biosensors based on chitosan-Co3O4-multiwall carbon nanotube modified glassy carbon electrode. Sensors Actuators, B. Chem., 200:83–91.
- [82] Dervisevic, M., Dervisevic, E., Şenel, M., (2018). Design of amperometric urea biosensor based on self-assembled monolayer of cystamine/PAMAM-grafted MWCNT/Urease. Sensors Actuators B. Chem., 254:93–101.
- [83] Karimi-Maleh, H., Tahernejad-Javazmi, F., Atar, N., Yola, M.L., Gupta, V.K., Ensafi, A.A., (2015). A Novel DNA Biosensor Based on a Pencil Graphite Electrode Modified with Polypyrrole/Functionalized Multiwalled Carbon Nanotubes for Determination of 6-Mercaptopurine Anticancer Drug. Ind. Eng. Chem. Res., 54:3634–3639.
- [84] Dinçkaya E, Kinik Ö, Sezgintürk MK, Altuĝ Ç, Akkoca A (2011). Development of an impedimetric aflatoxin M1 biosensor based on a DNA probe and gold nanoparticles. Biosens. Bioelectron, 26:3806–3811.
- [85] Yola, M.L., Eren, T., Atar, N., (2014). A novel and sensitive electrochemical DNA biosensor based on Fe@Au nanoparticles decorated graphene oxide. Electrochim. Acta, 125:38– 47.
- [86] Yola, M.L., Eren, T., Atar, N., (2014). Molecularly imprinted electrochemical biosensor based on Fe@Au nanoparticles involved in 2-aminoethanethiol functionalized multi-walled carbon nanotubes for sensitive determination of cefexime in human plasma. Biosens. Bioelectron, 60:277–285.
- [87] Ozalp, V.C., Bayramoglu, G., Erdem, Z., Arica, M.Y., (2015). Pathogen detection in complex samples by quartz crystal microbalance sensor coupled to aptamer functionalized core- shell type magnetic separation. Anal Chim. Acta, 853:533– 540.
- [88] Parlak, O., İncel, A., Uzun, L., Turner, A.P.F., Tiwari, A., (2017). Structuring Au nanoparticles on two-dimensional MoS2 nanosheets for electrochemical glucose biosensors. Biosens. Bioelectron., 89:545–550.
- [89] Koskun, Y., Şavk, A., Şen, B., Şen, F., (2018). Highly sensitive glucose sensor based on monodisperse palladium nickel/ activated carbon nanocomposites. Anal. Chim. Acta, 1010:37–43.
- [90] Şenel, M., Nergiz, C., (2012). Novel amperometric glucose biosensor based on covalent immobilization of glucose oxidase on poly(pyrrole propylic acid)/Au nanocomposite. Curr. Appl. Phys., 12:1118–1124.
- [91] Kilic, T., Topkaya, S.N., Ariksoysal, D.O., Ozsoz, M., Ballar, P., Erac, Y., Gozen, O., (2012). Electrochemical based detection of microRNA, mir21 in breast cancer cells. Biosens. Bioelectron, 38:195–201.
- [92] Kilic, T., Topkaya, S.N., Ozsoz, M., (2013). A new insight into electrochemical microRNA detection: A molecular caliper, p19 protein. Biosens. Bioelectron. 48:165–171.
- [93] Atar, N., Eren, T., Yola, M.L., (2015). A molecular imprinted SPR biosensor for sensitive determination of citrinin in red yeast rice. Food. Chem., 184:7–11.
- [94] Kesik, M., Akbulut, H., Söylemez, S., Cevher, Ş.C., Hızalan, G., Udum, Y.A., Endo, T., Yamada, S., Çırpan, A., Yağcı, Y., (2014). Synthesis and characterization of conducting polymers containing polypeptide and ferrocene side chains as ethanol biosensors. Polym. Chem., 5:6295–6306.
- [95] Yilmaz Ö, Demirkol DO, Gülcemal S, Kilinç A, Timur S, Çetinkaya B (2012). Chitosan-ferrocene film as a platform for flow injection analysis applications of glucose oxidase and Gluconobacter oxydans biosensors. Colloids Surfaces B. Biointerfaces, 100:62–68.
- [96] Perk, O.Y., Şeşen, M., Gozuacik., D., Koşar, A., (2012). Kidney stone erosion by micro scale hydrodynamic cavitation and consequent kidney stone treatment. Ann. Biomed. Eng., 40:1895–1902.
- [97] Oflaz, H., Baran, O., (2014). A new medical device to measure a stiffness of soft materials. Acta Bioeng. Biomech., 16:125–131.
- [98] Yen, P-L., Chen, D-R., Yeh, K-T., Chu, P-Y. (2008). Lateral exploration strategy for differentiating the stiffness ratio of an inclusion in soft tissue. Med. Eng. Phys., 30:1013–1019.
- [99] Subasi, A., Gursoy, M.I., (2010). EEG signal classification using PCA, ICA, LDA and support vector machines. Expert Syst. Appl., 37:8659–8666.
- [100] Acar, E., Dunlavy, DM., Kolda, T.G., Mørup, M., (2011). Scalable tensor factorizations for incomplete data. Chemom. Intell. Lab. Syst., 106:41–56.
- [101] Ince, T., Kiranyaz, S., Gabbouj, M., (2009). A generic and robust system for automated patient-specific classification of ECG signals. IEEE Trans. Biomed. Eng., 56:1415–1426.
- [102] Kiranyaz, S., Ince, T., Gabbouj, M., (2016). Real-Time Patient- Specific ECG Classification by 1-D Convolutional Neural Networks. IEEE Trans. Biomed. Eng., 63:664–675.
- [103] Tabar, Y.R., Halici, U., (2016). A novel deep learning approach for classification of EEG motor imagery signals. J. Neural Eng., 14:16003.
- [104] Alkan, A., Günay, M., (2012). Identification of EMG signals using discriminant analysis and SVM classifier. Expert. Syst. Appl., 39:44–47.
- [105] Dincer, F., Karaaslan, M., Unal, E., Sabah, C., (2013). Dual- band polarization independent meta-material absorber based on omega resonator and octastarstrip configuration. Prog. Electromagn. Res., 141:219–231.
- [106] Sabah, C., Dincer, F., Karaaslan, M., Unal, E., Akgol, O., Demirel, E., (2014). Perfect metamaterial absorber with polarization and incident angle independencies based on ring and cross-wire resonators for shielding and a sensor application. Opt. Commun., 322:137–142.
- [107] Ergen, B., Tatar, Y., Gulcur, H.O., (2012). Time-frequency analysis of phonocardiogram signals using wavelet transform: A comparative study. Comput. Methods, Biomech. Biomed. Engin., 15:371–381.
- [108] Gurun, G., Zahorian, J.S., Sisman, A., Karaman, M., Hasler, P..E, Degertekin, L.F., (2012). An analog integrated circuit beamformer for high-frequency medical ultrasound imaging. IEEE Trans. Biomed. Circuits Syst. 6:454–467.
- [109] Kerse, C., Kalaycıoğlu, H., Elahi, P., Çetin, B., Kesim, D.K., Akçaalan, Ö., Yavaş, S., Aşık, M.D., Öktem, B., Hoogland, H., (2016). Ablation-cooled material removal with ultrafast bursts of pulses. Nature, 537:84.
- [110] Kucukgul, C., Ozler, S.B., Inci, I., Karakas, E., Irmak, S., Gozuacik, D., Taralp, A., Koc, B., (2015). 3D bioprinting of biomimetic aortic vascular constructs with self-supporting cells. Biotechnol Bioeng 112:811–821.
APA | Karamanlioglu M (2019). A Review of Biomedical Engineering Research in Turkey During the Period 2008-2018. , 316 - 327. 10.7240/jeps.578024 |
Chicago | Karamanlioglu Mehlika A Review of Biomedical Engineering Research in Turkey During the Period 2008-2018. (2019): 316 - 327. 10.7240/jeps.578024 |
MLA | Karamanlioglu Mehlika A Review of Biomedical Engineering Research in Turkey During the Period 2008-2018. , 2019, ss.316 - 327. 10.7240/jeps.578024 |
AMA | Karamanlioglu M A Review of Biomedical Engineering Research in Turkey During the Period 2008-2018. . 2019; 316 - 327. 10.7240/jeps.578024 |
Vancouver | Karamanlioglu M A Review of Biomedical Engineering Research in Turkey During the Period 2008-2018. . 2019; 316 - 327. 10.7240/jeps.578024 |
IEEE | Karamanlioglu M "A Review of Biomedical Engineering Research in Turkey During the Period 2008-2018." , ss.316 - 327, 2019. 10.7240/jeps.578024 |
ISNAD | Karamanlioglu, Mehlika. "A Review of Biomedical Engineering Research in Turkey During the Period 2008-2018". (2019), 316-327. https://doi.org/10.7240/jeps.578024 |
APA | Karamanlioglu M (2019). A Review of Biomedical Engineering Research in Turkey During the Period 2008-2018. International journal of advances in engineering and pure sciences (Online), 31(4), 316 - 327. 10.7240/jeps.578024 |
Chicago | Karamanlioglu Mehlika A Review of Biomedical Engineering Research in Turkey During the Period 2008-2018. International journal of advances in engineering and pure sciences (Online) 31, no.4 (2019): 316 - 327. 10.7240/jeps.578024 |
MLA | Karamanlioglu Mehlika A Review of Biomedical Engineering Research in Turkey During the Period 2008-2018. International journal of advances in engineering and pure sciences (Online), vol.31, no.4, 2019, ss.316 - 327. 10.7240/jeps.578024 |
AMA | Karamanlioglu M A Review of Biomedical Engineering Research in Turkey During the Period 2008-2018. International journal of advances in engineering and pure sciences (Online). 2019; 31(4): 316 - 327. 10.7240/jeps.578024 |
Vancouver | Karamanlioglu M A Review of Biomedical Engineering Research in Turkey During the Period 2008-2018. International journal of advances in engineering and pure sciences (Online). 2019; 31(4): 316 - 327. 10.7240/jeps.578024 |
IEEE | Karamanlioglu M "A Review of Biomedical Engineering Research in Turkey During the Period 2008-2018." International journal of advances in engineering and pure sciences (Online), 31, ss.316 - 327, 2019. 10.7240/jeps.578024 |
ISNAD | Karamanlioglu, Mehlika. "A Review of Biomedical Engineering Research in Turkey During the Period 2008-2018". International journal of advances in engineering and pure sciences (Online) 31/4 (2019), 316-327. https://doi.org/10.7240/jeps.578024 |