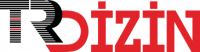
Glucose metabolism and oncogenes in cancer
Yıl: 2021 Cilt: 28 Sayı: 8 Sayfa Aralığı: 1605 - 1610 Metin Dili: İngilizce DOI: 10.5455/annalsmedres.2020.09.903 İndeks Tarihi: 11-11-2021
Glucose metabolism and oncogenes in cancer
Öz: Cancer cells utilize glucose quite differently from regular cells as cancer cells metabolize glucose more in aerobic glycolysis rather than in oxidative phosphorylation. Whereas aerobic glycolysis is less effective in the metabolism than oxidative phosphorylation. This review aims to explain the mechanisms of cancer metabolism and recent findings related to the subject. There are excessive glycolysis and glucose transport in tumor cells, this situation as known Warburg effect. Mitochondrial impairment, hypoxia, oncogenic signals, and defected metabolic enzymes are mechanisms of this cancer metabolism. Results of increased glycolysis are quick production of ATP and intermediates for biosynthetic pathways and occur acidic cell environment. The oncogenes, hypoxiainducible factor (HIF), serine/threonine kinase Akt, K-ras, c-myc, AMP-activated protein kinase (AMPK) and p53 have important roles in cancer metabolism. HIF, Akt, K-ras, c-myc, AMPK, and p53 are important oncogenes in cancer metabolism. The differences in metabolism of cancer cells are important targets for new treatment methods.
Anahtar Kelime: Belge Türü: Makale Makale Türü: Derleme Erişim Türü: Erişime Açık
- 1. Murray RK, Davis JC. Harper’s Illustrated Biochemistry 30th Edition. Molecular Physiology 2014:738-39
- 2. Warburg O. On the origin of cancer cells. Science1956;123:309-14.
- 3. Voet D, Voet JG. Pyruvate Dehydrogenase Multienzyme Complex. Biochem 2nd Ed 1995;241.
- 4. Liberti M V., Locasale JW. The Warburg Effect: How Does it Benefit Cancer Cells? Trends Biochem Sci 2016;41:211-8.
- 5. Farwell MD, Pryma DA, Mankoff DA. PET/CT imaging in cancer: Current applications and future directions. Cancer 2014 ;120:3433-45.
- 6. López-Ríos F, Sánchez-Aragó M, García-Garía Eet al. Loss of the mitochondrial bioenergetic capacity underlies the glucose avidity of carcinomas. Cancer Res 2007;67:9013-7.
- 7. Heiden MGV, Cantley LC, Thompson CB. Understanding the warburg effect: The metabolic requirements of cell proliferation. Science 2009;324:1029-33.
- 8. Gatenby RA, Gillies RJ. Why do cancers have high aerobic glycolysis? Nature Reviews Cancer. 2004;4:891-9.
- 9. Brand KA, Hermfisse U. Aerobic glycolysis by proliferating cells: a protective strategy against reactive oxygen species 1 . FASEB J 1997;11:388-95.
- 10. Yecies JL, Manning BD. Chewing the Fat on Tumor Cell Metabolism. Cell 2010;140:28-30.
- 11. Nomura DK, Long JZ, Niessen S et al. Monoacylglycerol Lipase Regulates a Fatty Acid Network that Promotes Cancer Pathogenesis. Cell 2010;140:49-61.
- 12. Sawayama H, Ishimoto T, Watanabe M et al. High expression of glucose transporter 1 on primary lesions of esophageal squamous cell carcinoma is associated with hematogenous recurrence. Ann Surg Oncol 2014;21:1756-62.
- 13. Goodwin J, Neugent ML, Lee SYet al. The distinct metabolic phenotype of lung squamous cell carcinoma defines selective vulnerability to glycolytic inhibition. Nat Commun 2017;8:15503.
- 14. Ebert BL, Firth JD, Ratcliffe PJ. Hypoxia and mitochondrial inhibitors regulate expression of glucose transporter-1 via distinct cis-acting sequences. J Biol Chem 1995; 270:29083-9.
- 15. Haber RS, Rathan A, Weiser KR et al. GLUT1 glucose transporter expression in colorectal carcinoma: A marker for poor prognosis. Cancer 1998;83:34-40.
- 16. Goos JACM, De Cuba EMV, Coupé VMH et al. Glucose transporter 1 (SLC2A1) and vascular endothelial growth factor A (VEGFA) predict survival after resection of colorectal cancer liver metastasis. Ann Surg 2016;263:138-45.
- 17. Hiyoshi Y, Watanabe M, Imamura Y et al. The relationship between the glucose transporter type 1 expression and 18F-fluorodeoxyglucose uptake in esophageal squamous cell carcinoma. Oncology 2009;76:286-92.
- 18. Yun J, Mullarky E, Lu C et al. Vitamin C selectively kills KRAS and BRAF mutant colorectal cancer cells by targeting GAPDH. Science 2015;350:1391-6.
- 19. Van Heerden JH, Wortel MT, Bruggeman FJet al. Lost in transition: Start-up of glycolysis yields subpopulations of nongrowing cells. Science 2014;343:1245114.
- 20. Hitosugi T, Kang S, Vander Heiden MGet al. Tyrosine phosphorylation inhibits PKM2 to promote the warburg effect and tumor growth. Sci Signal 2009;2:73.
- 21. Cervera AM, Apostolova N, Crespo FLet al. Cells silenced for SDHB expression display characteristic features of the tumor phenotype. Cancer Res 2008;68:4058-67.
- 22. Baysal BE. A recurrent stop-codon mutation in succinate dehydrogenase subunit B gene in normal peripheral blood and childhood T-cell acute leukemia. PLoS One. 2007;2:e436.
- 23. Tomlinson IPM, Alam NA, Rowan AJ et al. Germline mutations in FH predispose to dominantly inherited uterine fibroids, skin leiomyomata and papillary renal cell cancer the multiple leiomyoma consortium. Nat Genet 2002;30:406-10.
- 24. Lee HC, Wei YH. Mitochondrial DNA instability and metabolic shift in human cancers. International Journal of Molecular Sciences 2009;10:674-701.
- 25. Pouysségur J, Dayan F, Mazure NM. Hypoxia signalling in cancer and approaches to enforce tumour regression. Nature 2006;441:437-43.
- 26. Smirnova NA, Hushpulian DM, Speer REet al. Catalytic mechanism and substrate specificity of HIF prolyl hydroxylases. Biochemistry (Moscow) 2012;77:1108- 19.
- 27. Papandreou I, Cairns RA, Fontana Let al. HIF1 mediates adaptation to hypoxia by actively downregulating mitochondrial oxygen consumption. Cell Metab 2006;3:187-97.
- 28. Rempel A, Mathupala SP, Griffin CA et al. Glucose catabolism in cancer cells: Amplification of the gene encoding type II hexokinase. Cancer Res 1996;56:2468-71.
- 29. Lum JJ, Bui T, Gruber M et al. The transcription factor HIF-1 plays a critical role in the growth factordependent regulation of both aerobic and anaerobic glycolysis. Genes Dev 2007;21:1037-49.
- 30. Tang CM, Yu J. Hypoxia-inducible factor-1 as a therapeutic target in cancer. J Gastroenterol Hepatol 2013;28:401-5.
- 31. Zhou J, Huang S, Wang L et al. Clinical and prognostic significance of HIF-1α overexpression in oral squamous cell carcinoma: A meta-analysis. World J Surg Oncol 2017;15:104.
- 32. Elstrom RL, Bauer DE, Buzzai M et al. Akt stimulates aerobic glycolysis in cancer cells. Cancer Res 2004; 64:3892-9.
- 33. Barthel A, Okino ST, Liao J et al. Regulation of GLUT1 gene transcription by the serine/threonine kinase Akt1. J Biol Chem 1999;274:20281-6.
- 34. Bauer DE, Hatzivassiliou G, Zhao Fet al. ATP citrate lyase is an important component of cell growth and transformation. Oncogene 2005; 24:6314-22.
- 35. Yun J, Rago C, Cheong I et al. Glucose deprivation contributes to the development of KRAS pathway mutations in tumor cells. Science 2009;325:1555-9.
- 36. Dang C V., Le A, Gao P. MYC-induced cancer cell energy metabolism and therapeutic opportunities. Clin Cancer Res 2009;15:6479-83.
- 37. Wahlström T, Arsenian Henriksson M. Impact of MYC in regulation of tumor cell metabolism. Biochimica et Biophysica Acta - Gene Regulatory Mechanisms 2015;1849:563-9.
- 38. Stine ZE, Walton ZE, Altman BJ et al. MYC, metabolism, and cancer. Cancer Discovery 2015; 5:1024-39.
- 39. O’Connell BC, Cheung AF, Simkevich CP et al. A large scale genetic analysis of c-Myc-regulated gene expression patterns. J Biol Chem 2003;278:12563-73
- 40. Kim J, Zeller KI, Wang Y et al. Evaluation of Myc E-Box Phylogenetic Footprints in Glycolytic Genes by Chromatin Immunoprecipitation Assays. Mol Cell Biol 2004;24:5923-36.
- 41. Shim H, Dolde C, Lewis BCet al. c-Myc transactivation of LDH-A: Implications for tumor metabolism and growth. Proc Natl Acad Sci U S A. 1997;94:6658-63.
- 42. Osthus RC, Shim H, Kim S et al. Deregulation of glucose transporter 1 and glycolytic gene expression by c-Myc. J Biol Chem 2000; 275:21797-800.
- 43. Kahn BB, Alquier T, Carling Det al. AMP-activated protein kinase: Ancient energy gauge provides clues to modern understanding of metabolism. Cell Metabolism 2005;1:15-25.
- 44. Carling D. The AMP-activated protein kinase cascade - A unifying system for energy control. Trends in Biochemical Sciences 2004;29:18-24.
- 45. Vousden KH, Ryan KM. P53 and metabolism. Nature Reviews Cancer. 2009;9:691-700.
- 46. Schwartzenberg-Bar-Yoseph F, Armoni M, Karnieli E. The Tumor Suppressor p53 Down-Regulates Glucose Transporters GLUT1 and GLUT4 Gene Expression. Cancer Res 2004;64:2627-33.
- 47. Kawauchi K, Araki K, Tobiume Ket al. Activated p53 induces NF-κB DNA binding but suppresses its transcriptional activation. Biochem Biophys Res Commun 2008;372:137-41.
- 48. Webster NJG, Resnik JL, Reichart DBet al. Repression of the insulin receptor promoter by the tumor suppressor gene product p53: A possible mechanism for receptor overexpression in breast cancer. Cancer Res 1996;56:2781-8.
- 49. Boidot R, Vegran F, Meulle Aet al. Regulation of monocarboxylate transporter MCT1 expression by p53 mediates inward and outward lactate fluxes in tumors. Cancer Res 2012;72:939-48.
- 50. Matoba S, Kang JG, Patino WD et al. p53 regulates mitochondrial respiration. Science 2006;312:1650-3.
- 51. Bensaad K, Tsuruta A, Selak MA et al. TIGAR, a p53- Inducible Regulator of Glycolysis and Apoptosis. Cell 2006;126:107-20.
- 52. Kondoh H, Lleonart ME, Gil J et al. Glycolytic enzymes can modulate cellular life span. Cancer Res 2005;65:177-85.
- 53. Feng Z, Zhang H, Levine AJ et al. The coordinate regulation of the p53 and mTOR pathways in cells. Proc Natl Acad Sci U S A. 2005;102:8204-9.
- 54. Budanov A V., Karin M. p53 Target Genes Sestrin1 and Sestrin2 Connect Genotoxic Stress and mTOR Signaling. Cell 2008;134:451-60.
- 55. Kim HR, Roe JS, Lee JEet al. P53 regulates glucose metabolism by miR-34a. Biochem Biophys Res Commun 2013;437:225-31.
- 56. Yeo SY, Itahana Y, Guo AKet al. Transglutaminase 2 contributes to a TP53- induced autophagy program to prevent oncogenic transformation. Elife 2016;5:e07101.
- 57. Gerweck LE, Seetharaman K. Cellular pH gradient in tumor versus normal tissue: Potential exploitation for the treatment of cancer. Cancer Res 1996;56:1194-8.
- 58. Ganapathy-Kanniappan S, Kunjithapatham R, Geschwind JF. Anticancer efficacy of the metabolic blocker 3-bromopyruvate: Specific molecular targeting. Anticancer Res 2013;33:13-20.
- 59. Pang YY, Wang T, Chen FY et al. Glycolytic inhibitor 2-deoxy-d-glucose suppresses cell proliferation and enhances methylprednisolone sensitivity in nonHodgkin lymphoma cells through down-regulation of HIF-1α and c-MYC. Leuk Lymphoma 2015;56:1821- 30.
- 60. Casinelli G, LaRosa J, Sharma Met al. N-Myc overexpression increases cisplatin resistance in neuroblastoma via deregulation of mitochondrial dynamics. Cell Death Discov 2016;2:16082.
APA | MERTOĞLU C (2021). Glucose metabolism and oncogenes in cancer. , 1605 - 1610. 10.5455/annalsmedres.2020.09.903 |
Chicago | MERTOĞLU Cuma Glucose metabolism and oncogenes in cancer. (2021): 1605 - 1610. 10.5455/annalsmedres.2020.09.903 |
MLA | MERTOĞLU Cuma Glucose metabolism and oncogenes in cancer. , 2021, ss.1605 - 1610. 10.5455/annalsmedres.2020.09.903 |
AMA | MERTOĞLU C Glucose metabolism and oncogenes in cancer. . 2021; 1605 - 1610. 10.5455/annalsmedres.2020.09.903 |
Vancouver | MERTOĞLU C Glucose metabolism and oncogenes in cancer. . 2021; 1605 - 1610. 10.5455/annalsmedres.2020.09.903 |
IEEE | MERTOĞLU C "Glucose metabolism and oncogenes in cancer." , ss.1605 - 1610, 2021. 10.5455/annalsmedres.2020.09.903 |
ISNAD | MERTOĞLU, Cuma. "Glucose metabolism and oncogenes in cancer". (2021), 1605-1610. https://doi.org/10.5455/annalsmedres.2020.09.903 |
APA | MERTOĞLU C (2021). Glucose metabolism and oncogenes in cancer. Annals of Medical Research, 28(8), 1605 - 1610. 10.5455/annalsmedres.2020.09.903 |
Chicago | MERTOĞLU Cuma Glucose metabolism and oncogenes in cancer. Annals of Medical Research 28, no.8 (2021): 1605 - 1610. 10.5455/annalsmedres.2020.09.903 |
MLA | MERTOĞLU Cuma Glucose metabolism and oncogenes in cancer. Annals of Medical Research, vol.28, no.8, 2021, ss.1605 - 1610. 10.5455/annalsmedres.2020.09.903 |
AMA | MERTOĞLU C Glucose metabolism and oncogenes in cancer. Annals of Medical Research. 2021; 28(8): 1605 - 1610. 10.5455/annalsmedres.2020.09.903 |
Vancouver | MERTOĞLU C Glucose metabolism and oncogenes in cancer. Annals of Medical Research. 2021; 28(8): 1605 - 1610. 10.5455/annalsmedres.2020.09.903 |
IEEE | MERTOĞLU C "Glucose metabolism and oncogenes in cancer." Annals of Medical Research, 28, ss.1605 - 1610, 2021. 10.5455/annalsmedres.2020.09.903 |
ISNAD | MERTOĞLU, Cuma. "Glucose metabolism and oncogenes in cancer". Annals of Medical Research 28/8 (2021), 1605-1610. https://doi.org/10.5455/annalsmedres.2020.09.903 |