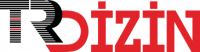
Thermal stresses in SOFC stacks: the role of mismatch among thermal conductivity of adjacent components
Yıl: 2021 Cilt: 45 Sayı: 3 Sayfa Aralığı: 719 - 736 Metin Dili: İngilizce DOI: 10.3906/kim-2011-48 İndeks Tarihi: 28-06-2022
Thermal stresses in SOFC stacks: the role of mismatch among thermal conductivity of adjacent components
Öz: Generating power from renewable biogas in solid oxide fuel cells (SOFCs) is an environment-friendly, efficient, and promising energy conversion process. Biogas can be used in SOFCs via a reforming process for which dry reforming is more suitable as the reforming agent exists in the biogas mixture. Biogas can be directly reformed to $H_2$ -rich fuel stream in the anode chamber of a SOFC by the heat released during power generation. Exploiting the heat and water produced in the SOFC for internal reforming of biogas makes the energy conversion process very efficient; however, various challenges are reported. Thus, indirect internal reforming is opted for which a separate reforming domain is required. In an indirect internal reformer operating at usual conditions, dry reforming rate is quite high in the inlet and it decreases steeply toward the fuel outlet. Great temperature gradients develop over the reformer, since the dry reforming reaction is strongly endothermic. The abruptly varying rate of the reforming reaction affects the temperature fields in the adjacent components of SOFC and hence intolerable thermal stresses emerge on the SOFC components. In our preceding study, we graded the reforming domain, homogenized the temperature profile over the reforming domain, and executed performance and durability experiments. However, most of the experiments failed due to fracturing SOFC components hinting at existence of thermal stresses. In that study, we focused on minimizing the temperature gradients within the reforming domain; namely, we neglected the other processes. To eliminate the thermal stresses, we modeled the entire module of SOFC equipped with a reformer featuring a graded reforming domain. We found that the mismatch between the thermal conductivities of the adjacent module components is the major reason for the thermal stresses. When the mismatch is eliminated, thermal stresses disappear even if the reforming domain is not graded.
Anahtar Kelime: Belge Türü: Makale Makale Türü: Araştırma Makalesi Erişim Türü: Erişime Açık
- [1] Dicks AL. Hydrogen generation from natural gas for the fuel cell systems of tomorrow. Journal of Power Sources 1996; 61: 113-124.
- [2] Shiratori Y, Ijichi T, Oshima T, Sasaki K. Internal reforming SOFC running on biogas. International Journal of Hydrogen Energy 2010; 35: 7905-7912.
- [3] Shiratori Y, Ogura T, Nakajima H, Sakamoto M, Takahashi Y et al. Study on paper-structured catalyst for direct internal reforming SOFC fueled by the mixture of CH4 and CO2. International Journal of Hydrogen Energy 2013; 38: 10542-10551.
- [4] Shiratori Y, Quang-Tuyen T, Sasaki K. Performance enhancement of biodiesel fueled SOFC using paperstructured catalyst. International Journal of Hydrogen Energy 2013; 38: 9856-9866.
- [5] Shiratori Y, Oshima T, Sasaki K. Feasibility of direct-biogas SOFC. International Journal of Hydrogen Energy 2008; 33: 6316-6321.
- [6] Alves HJ, Junior CB, Niklevicz RR, Frigo EP, Frigo MS. Overview of hydrogen production technologies from biogas and the applications in fuel cells. International Journal of Hydrogen Energy 2013; 38: 5215-5225.
- [7] Huang J, Crookes RJ. Assessment of simulated biogas as a fuel for the spark ignition engine. Fuel 1998; 77: 1793-1801.
- [8] Achenbach E, Riensche E. Methane/steam reforming kinetics for solid oxide fuel cells. Journal of Power Sources 1994; 52: 283-288.
- [9] Nobrega SD, Gelin P, Georges S, Steil MC, Augusto BL et al. A fuel-flexible solid oxide fuel cell operating in gradual internal reforming. Journal of the Electrochemical Society 2014; 161 (3): F354-F359.
- [10] Susumu N, Momma A, Kato T, Kasuga Y. Numerical analysis of output characteristics of tubular SOFC with internal reformer. Journal of Power Sources 2001; 101: 60-71.
- [11] Brus G, Szmyd JS. Numerical modelling of radiative heat transfer in an internal indirect reforming-type SOFC. Journal of Power Sources 2008; 181: 8-16.
- [12] Shiratori Y, Sakamoto M, Uchida T, Le H, Quang-Tuyen T et al. Hydrotalcite-dispersed paper-structured catalyst for the dry reforming of methane. International Journal of Hydrogen Energy 2015; 40: 10807-10815.
- [13] Shiratori Y, Sakamoto M. Performance improvement of direct internal reforming solid oxide fuel cell fuelled by H2S-contaminated biogas with paper-structured catalyst technology. Journal of Power Sources 2016; 332: 170-179.
- [14] Aydın Ö, Nakajima H, Kitahara T. Current and temperature distributions in-situ acquired by electrodesegmentation along a microtubular solid oxide fuel cell operating with syngas. Journal of Power Sources 2015; 293: 1053-1061.
- [15] Kirtley JD, Steinhurst DA, Owrutsky JC, Pomfret MB, Walker RA. In situ optical studies of methane and simulated biogas oxidation on high temperature solid oxide fuel cell anodes. Physical Chemistry Chemical Physics 2014; 16: 227.
- [16] Lanzini A, Leone P. Experimental investigation of direct internal reforming of biogas in solid oxide fuel cells. International Journal of Hydrogen Energy 2010; 35: 2463-2476.
- [17] Tran DL, Tuyen-Tran Q, Sakamoto M, Sasaki K, Shiratori Y. Modelling of ch4 multiple-reforming within the ni-ysz anode of a solid oxide fuel cell. Journal of Power Sources 2017; 359: 507-519.
- [18] Saunders J, Davy M. In-situ studies of gas phase composition and anode surface temperature through a model DIR-SOFC steam-methane reformer at 973.15 K. International Journal of Hydrogen Energy 2013; 38: 13762-13773.
- [19] Aydın Ö, Kubota A, Tran DL, Sakamoto M, Shiratori Y. Designing graded catalytic domain to homogenize temperature distribution while dry reforming of ch4. International Journal of Hydrogen Energy 2018; 43: 17431-17443.
- [20] Nishino T, Iwai H, Suzuki K. Comprehensive numerical modeling and analysis of a cell-based indirect internal reforming tubular SOFC. Journal of Fuel Cell Science and Technology 2006; 101: 33-34.
- [21] Pajak M, Mozdzierz M, Chalusiak B, Kimijima S, Szmyd JS et al. A numerical analysis of heat and mass transfer processes in a macro-patterned methane/steam reforming reactor. International Journal of Hydrogen Energy 2018; 43: 20474-20487.
- [22] Aydın Ö, Matsumoto G, Kubota A, Tran DL, Sakamoto M et al. Performance and durability of onecell module of biogas-utilizing SOFC equipped with graded indirect internal reformer. Journal of the Electrochemical Society 2020; 167 (6): 064512.
- [23] Serincan MF, Pasaogullari U, Singh P. Controlling reformation rate for a more uniform temperature distribution in an internal methane steam reforming solid oxide fuel cell. Journal of Power Sources 2020; 468: 228310.
- [24] Yaws CL. Yaws Handbook of Thermodynamic and Physical Properties of Chemical Compounds. Texas, USA:Knovel / McGraw-Hill, 2003.
- [25] Aydın Ö, Nakajima H, Kitahara T. Reliability of the numerical SOFC models for estimating the spatial current and temperature variations. International Journal of Hydrogen Energy 2016; 41: 15311-15324.
- [26] Fuller EN, Schettler PD, Giddings JC. A new method for prediction of binary gas-phase diffusion coefficients. Industrial & Engineering Chemistry Research 1966; 58: 18-27.
- [27] Todd B, Young J. Thermodynamic and transport properties of gases for use in solid oxide fuel cell modelling. Journal of Power Sources 2002; 110 (1): 186-200.
- [28] Serincan MF, Pasaogullari U, Sammes NM. Computational thermal-fluid analysis of a microtubular solid oxide fuel cell. Journal of the Electrochemical Society 2008; 155 (11): B1117-B1127.
- [29] Afshar R, Cogley AC, Saxena SC. Thermal conductivity of methane at atmospheric pressure in the temperature range of 360-1275 K. Journal of Heat Transfer 1980; 102 (1): 163-167.
- [30] Gupta G, Saxena S. Thermal conductivity of carbon dioxide in the temperature range 100°C to 1075°C. Molecular Physics 1970; 19 (6): 871-880.
- [31] Millat J, Wakeham WA. The thermal conductivity of nitrogen and carbon monoxide in the limit of zero density. Journal of Physical and Chemical Reference Data 1989; 18: 565-581.
- [32] Patcharavorachot Y, Arpornwichanop A, Chuachuensuk A. Electrochemical study of planar solid oxide fuel cell: role of support structures. Journal of Power Sources 2008; 177: 254-261.
- [33] Janardhanan VM, Deutschman O. Numerical study of mass and heat transport in solid oxide fuel cells running on humidified methane. Chemical Engineering Science 2007; 62: 5473-5486.
APA | AYDIN Ö, MATSUMOTO G, SHIRATORI Y (2021). Thermal stresses in SOFC stacks: the role of mismatch among thermal conductivity of adjacent components. , 719 - 736. 10.3906/kim-2011-48 |
Chicago | AYDIN Özgür,MATSUMOTO Go,SHIRATORI Yusuke Thermal stresses in SOFC stacks: the role of mismatch among thermal conductivity of adjacent components. (2021): 719 - 736. 10.3906/kim-2011-48 |
MLA | AYDIN Özgür,MATSUMOTO Go,SHIRATORI Yusuke Thermal stresses in SOFC stacks: the role of mismatch among thermal conductivity of adjacent components. , 2021, ss.719 - 736. 10.3906/kim-2011-48 |
AMA | AYDIN Ö,MATSUMOTO G,SHIRATORI Y Thermal stresses in SOFC stacks: the role of mismatch among thermal conductivity of adjacent components. . 2021; 719 - 736. 10.3906/kim-2011-48 |
Vancouver | AYDIN Ö,MATSUMOTO G,SHIRATORI Y Thermal stresses in SOFC stacks: the role of mismatch among thermal conductivity of adjacent components. . 2021; 719 - 736. 10.3906/kim-2011-48 |
IEEE | AYDIN Ö,MATSUMOTO G,SHIRATORI Y "Thermal stresses in SOFC stacks: the role of mismatch among thermal conductivity of adjacent components." , ss.719 - 736, 2021. 10.3906/kim-2011-48 |
ISNAD | AYDIN, Özgür vd. "Thermal stresses in SOFC stacks: the role of mismatch among thermal conductivity of adjacent components". (2021), 719-736. https://doi.org/10.3906/kim-2011-48 |
APA | AYDIN Ö, MATSUMOTO G, SHIRATORI Y (2021). Thermal stresses in SOFC stacks: the role of mismatch among thermal conductivity of adjacent components. Turkish Journal of Chemistry, 45(3), 719 - 736. 10.3906/kim-2011-48 |
Chicago | AYDIN Özgür,MATSUMOTO Go,SHIRATORI Yusuke Thermal stresses in SOFC stacks: the role of mismatch among thermal conductivity of adjacent components. Turkish Journal of Chemistry 45, no.3 (2021): 719 - 736. 10.3906/kim-2011-48 |
MLA | AYDIN Özgür,MATSUMOTO Go,SHIRATORI Yusuke Thermal stresses in SOFC stacks: the role of mismatch among thermal conductivity of adjacent components. Turkish Journal of Chemistry, vol.45, no.3, 2021, ss.719 - 736. 10.3906/kim-2011-48 |
AMA | AYDIN Ö,MATSUMOTO G,SHIRATORI Y Thermal stresses in SOFC stacks: the role of mismatch among thermal conductivity of adjacent components. Turkish Journal of Chemistry. 2021; 45(3): 719 - 736. 10.3906/kim-2011-48 |
Vancouver | AYDIN Ö,MATSUMOTO G,SHIRATORI Y Thermal stresses in SOFC stacks: the role of mismatch among thermal conductivity of adjacent components. Turkish Journal of Chemistry. 2021; 45(3): 719 - 736. 10.3906/kim-2011-48 |
IEEE | AYDIN Ö,MATSUMOTO G,SHIRATORI Y "Thermal stresses in SOFC stacks: the role of mismatch among thermal conductivity of adjacent components." Turkish Journal of Chemistry, 45, ss.719 - 736, 2021. 10.3906/kim-2011-48 |
ISNAD | AYDIN, Özgür vd. "Thermal stresses in SOFC stacks: the role of mismatch among thermal conductivity of adjacent components". Turkish Journal of Chemistry 45/3 (2021), 719-736. https://doi.org/10.3906/kim-2011-48 |