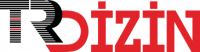
Mitokondriyal Disfonksiyonun Koroner Arter Hastalığı Üzerine Etkisi - Bölüm II
Yıl: 2023 Cilt: 51 Sayı: 3 Sayfa Aralığı: 202 - 211 Metin Dili: Türkçe DOI: 10.5543/tkda.2022.07282 İndeks Tarihi: 19-05-2023
Mitokondriyal Disfonksiyonun Koroner Arter Hastalığı Üzerine Etkisi - Bölüm II
Öz: Koroner arter hastalığının (KAH), temel nedeni olan aterosklerozun patogenezinde moleküler süreçlere ek olarak moleküler süreçler sonucunda işlevleri değişen organeller de yer almaktadır. Yakın zamanda mitokondri organelinin KAH patogenezindeki rolü araştırmacıların dikkatini çekmiştir. Mitokondri; oksijenli solunum, enerji üretimi ve hücre metabolizmasında düzenley- ici rolü olan, kendi genomuna sahip bir hücre organelidir. Hücrelerde mitokondri sayısı din- amik olarak değişmekte, işlevlerine ve enerji ihtiyacına göre her dokuda ve her hücrede farklı sayıda bulunmaktadır. Oksidatif stres mitokondriyal genomda ve mitokondriyal biyogenezde değişikliğe yol açarak mitokondriyal işlev bozukluğuna neden olur. Kardiyovasküler sistemde disfonksiyonel mitokondri popülasyonu KAH süreciyle ve hücre ölüm mekanizmaları ile yakından ilişkilidir. Ateroskleroz sürecinde yer alan moleküler değişimlere eşlik eden değişen mitokondri (dis)fonksiyonunun yakın gelecekte KAH’ın yeni terapötik hedefleri arasında yer alacağı düşünülmektedir.
Anahtar Kelime: Effect of Mitochondrial Dysfunction on Coronary Artery Disease - Part 2
Öz: Organelles whose functions change as a result of molecular processes are involved in the pathogenesis of atherosclerosis, which is the main cause of coronary artery disease, in addition to molecular processes. Recently, the role of mitochondria in the pathogenesis of coronary artery disease has attracted the attention of researchers. Mitochondria is a cell organelle with its own genome that plays a regulatory role in aerobic respiration, energy production, and cell metabolism. The number of mitochondria in cells changes dynamically, and there are differ- ent numbers of mitochondria in every tissue and every cell, depending on their function and energy needs. Oxidative stress causes mitochondrial dysfunction by leading to alterations in the mitochondrial genome and mitochondrial biogenesis. The dysfunctional mitochondria popula- tion in the cardiovascular system is closely related to the coronary artery disease process and cell death mechanisms. It is thought that the altered mitochondria (dys)function accompany- ing the molecular changes in the atherosclerosis process will be among the new therapeutic targets of coronary artery disease in the near future.
Anahtar Kelime: Belge Türü: Makale Makale Türü: Derleme Erişim Türü: Erişime Açık
- 1. Xu G-e, Song J, Gokulnath P, Vulugundam G, Xiao J. DUSP7: an Unusual Player in Adverse Cardiac Remodeling. In: Springer; Berlin; 2022:1-2.
- 2. Malakar AK, Choudhury D, Halder B, Paul P, Uddin A, Chakraborty S. A review on coronary artery disease, its risk factors, and therapeu- tics. J Cell Physiol. 2019;234(10):16812-16823. [CrossRef]
- 3. Mallat Z, Corbaz A, Scoazec A, et al. Expression of interleukin-18 in human atherosclerotic plaques and relation to plaque instability. Circulation. 2001;104(14):1598-1603. [CrossRef]
- 4. Gimbrone MA, Jr, García-Cardeña G. Endothelial cell dysfunction and the pathobiology of atherosclerosis. Circ Res. 2016;118(4):620- 636. [CrossRef]
- 5. Cyr AR, Huckaby LV, Shiva SS, Zuckerbraun BS. Nitric oxide and endothelial dysfunction. Crit Care Clin. 2020;36(2):307-321.[CrossRef]
- 6. Bayir H. Reactive oxygen species. Crit Care Med. 2005;33(Suppl): S498-S501. [CrossRef]
- 7. Matés JM. Effects of antioxidant enzymes in the molecular control of reactive oxygen species toxicology. Toxicology. 2000;153(1-3): 83-104. [CrossRef]
- 8. Liou GY, Storz P. Reactive oxygen species in cancer. Free Radic Res. 2010;44(5):479-496. [CrossRef]
- 9. Suárez-Rivero JM, Pastor-Maldonado CJ, Povea-Cabello S, et al. From mitochondria to atherosclerosis: the inflammation path. Bio- medicines. 2021;9(3). [CrossRef]
- 10. Ernster L, Schatz G. Mitochondria: a historical review. J Cell Biol. 1981;91(3 Pt 2):227s-55s. [CrossRef]
- 11. Giacomello M, Pyakurel A, Glytsou C, Scorrano L. The cell biology of mitochondrial membrane dynamics. Nat Rev Mol Cell Biol. 2020;21(4):204-224. [CrossRef]
- 12. Cogliati S, Enriquez JA, Scorrano L. Mitochondrial cristae: where beauty meets functionality. Trends Biochem Sci. 2016;41(3):261- 273. [CrossRef]
- 13. Quintana-Cabrera R, Mehrotra A, Rigoni G, Soriano ME. Who and how in the regulation of mitochondrial cristae shape and function. Biochem Biophys Res Commun. 2018;500(1):94-101. [CrossRef]
- 14. Palade GE. The fine structure of mitochondria. Anat Rec. 1952; 114(3):427-451. [CrossRef]
- 15. Chinnery PF, Schon EA. Mitochondria. J Neurol Neurosurg Psychia- try. 2003;74(9):1188-1199. [CrossRef]
- 16. Taanman JW. The mitochondrial genome: structure, transcription, translation and replication. Biochim BiophysActa. 1999;1410(2):103- 123. [CrossRef]
- 17. Silva-Pinheiro P, Minczuk M. The potential of mitochondrial genome engineering. Nat Rev Genet. 2022;23(4):199-214. [CrossRef]
- 18. Abdul-Maksoud RS, Sediq AM, Kattaia A, et al. Serum miR-210 and miR-155 expression levels as novel biomarkers for rheumatoid arthritis diagnosis. Br J Biomed Sci. 2017;74(4):209-213. [CrossRef]
- 19. Robin ED, Wong R. Mitochondrial DNA molecules and virtual num- ber of mitochondria per cell in mammalian cells. J Cell Physiol. 1988;136(3):507-513. [CrossRef]
- 20. Chen H, Chan DC. Mitochondrial dynamics–fusion, fission, move- ment, and mitophagy–in neurodegenerative diseases. Hum Mol Genet. 2009;18(R2):R169-R176. [CrossRef]
- 21. Quan Y, Xin Y, Tian G, Zhou J, Liu X. Mitochondrial ROS-modulated mtDNA: A potential target for cardiac aging. Oxid Med Cell Longev. 2020;2020:9423593. [CrossRef]
- 22. Jornayvaz FR, Shulman GI. Regulation of mitochondrial biogenesis. Essays Biochem. 2010;47:69-84. [CrossRef]
- 23. Hock MB, Kralli A. Transcriptional control of mitochondrial biogen- esis and function. Annu Rev Physiol. 2009;71:177-203. [CrossRef]
- 24. Popov LD. Mitochondrial biogenesis: an update. J Cell Mol Med. 2020;24(9):4892-4899. [CrossRef]
- 25. Carling D. AMPK signalling in health and disease. Curr Opin Cell Biol. 2017;45:31-37. [CrossRef]
- 26. Kim J, Yang G, Kim Y, Kim J, Ha J. AMPK activators: mechanisms of action and physiological activities. Exp Mol Med. 2016;48(4):e224. [CrossRef]
- 27. Herzig S, Shaw RJ. AMPK: guardian of metabolism and mitochon- drial homeostasis. Nat Rev Mol Cell Biol. 2018;19(2):121-135. [CrossRef]
- 28. Jäger S, Handschin C, St-Pierre J, Spiegelman BM. AMP-activated protein kinase (AMPK) action in skeletal muscle via direct phospho- rylation of PGC-1α. Proc Natl Acad Sci U S A. 2007;104(29):12017- 12022. [CrossRef]
- 29. Nogueiras R, Habegger KM, Chaudhary N, et al. Sirtuin 1 and sirtuin 3: physiological modulators of metabolism. Physiol Rev. 2012;92(3):1479-1514. [CrossRef]
- 30. Tang BL. Sirt1 and the mitochondria. Mol Cells. 2016;39(2):87-95. [CrossRef]
- 31. Westermann B. Mitochondrial fusion and fission in cell life and death. Nat Rev Mol Cell Biol. 2010;11(12):872-884. [CrossRef]
- 32. Diaz F, Moraes CT. Mitochondrial biogenesis and turnover. Cell Cal- cium. 2008;44(1):24-35. [CrossRef]
- 33. Hall AR, Burke N, Dongworth RK, Hausenloy DJ. Mitochondrial fusion and fission proteins: novel therapeutic targets for combating cardiovascular disease. Br J Pharmacol. 2014;171(8):1890-1906. [CrossRef]
- 34. Youle RJ, van der Bliek AM. Mitochondrial fission, fusion, and stress. Science. 2012;337(6098):1062-1065. [CrossRef]
- 35. Knott AB, Bossy-Wetzel E. Impairing the mitochondrial fission and fusion balance: a new mechanism of neurodegeneration. Ann N Y Acad Sci. 2008;1147:283-292. [CrossRef]
- 36. Ong SB, Kalkhoran SB, Cabrera-Fuentes HA, Hausenloy DJ. Mito- chondrial fusion and fission proteins as novel therapeutic targets for treating cardiovascular disease. Eur J Pharmacol. 2015;763(A):104- 114. [CrossRef]
- 37. Green K, Brand MD, Murphy MP. Prevention of mitochondrial oxida- tive damage as a therapeutic strategy in diabetes. Diabetes. 2004; 53(S110)(suppl 1):S110-S118. [CrossRef]
- 38. Eckmann J, Eckert SH, Leuner K, Muller WE, Eckert GP. Mitochon- dria: mitochondrial membranes in brain ageing and neurodegenera- tion. Int J Biochem Cell Biol. 2013;45(1):76-80. [CrossRef]
- 39. Vogel S, Rath D, Lu J, Chatterjee M, Geisler T, Gawaz M. Elevated mitochondrial membrane potential of circulating monocyte–plate- let aggregates in patients with coronary heart disease. Int J Cardiol. 2015;181:135-137. [CrossRef]
- 40. Phaniendra A, Jestadi DB, Periyasamy L. Free radicals: properties, sources, targets, and their implication in various diseases. Indian J Clin Biochem. 2015;30(1):11-26. [CrossRef]
- 41. Adwas AA, Elsayed A, Azab A, Quwaydir F. Oxidative stress and antioxidant mechanisms in human body. J Appl Biotechnol Bioeng. 2019;6:43-47.
- 42. Jauniaux GJB. Oxidative stress. Best Pract Res Clin Obstet Gynaecol. 2011;25:28.
- 43. Jaganjac M, Milkovic L, Zarkovic N, Zarkovic K. Oxidative stress and regeneration. Free Radic Biol Med. 2022;181:154-165. [CrossRef]
- 44. Thirupathi A, de Souza CT. Multi-regulatory network of ROS: the interconnection of ROS, PGC-1 alpha, and AMPK-SIRT1 during exercise. J Physiol Biochem. 2017;73(4):487-494. [CrossRef]
- 45. Donato AJ, Pierce GL, Lesniewski LA, Seals DR. Role of NFkappaB in age-related vascular endothelial dysfunction in humans. Aging (Albany NY). 2009;1(8):678-680. [CrossRef]
- 46. Kattoor AJ, Pothineni NVK, Palagiri D, Mehta JL. Oxidative stress in atherosclerosis. Curr Atheroscler Rep. 2017;19(11):42. [CrossRef]
- 47. Duprez L, Wirawan E, Vanden Berghe T, Vandenabeele P. Major cell death pathways at a glance. Microbes Infect. 2009;11(13):1050- 1062. [CrossRef]
- 48. D'Arcy MS. Cell death: a review of the major forms of apoptosis, necrosis and autophagy. Cell Biol Int. 2019;43(6):582-592.[CrossRef]
- 49. Voss AK, Strasser A. The essentials of developmental apoptosis. F1000Res. 2020;9. [CrossRef]
- 50. Kist M, Vucic D. Cell death pathways: intricate connections and disease implications. EMBO J. 2021;40(5):e106700. [CrossRef]
- 51. Vakifahmetoglu-Norberg H, Zhivotovsky B. The unpredictable caspase-2: what can it do? Trends Cell Biol. 2010;20(3):150-159. [CrossRef]
- 52. Hotchkiss RS, Strasser A, McDunn JE, Swanson PE. Cell death. N Engl J Med. 2009;361(16):1570-1583. [CrossRef]
- 53. Mizushima N. Autophagy: process and function. Genes Dev. 2007;21(22):2861-2873. [CrossRef]
- 54. Rubinsztein DC, Mariño G, Kroemer G. Autophagy and aging. Cell . 2011;146(5):682-695. [CrossRef]
- 55. He H, Liu X, Lv L, et al. Calcineurin suppresses AMPK-dependent cytoprotective autophagy in cardiomyocytes under oxidative stress. Cell Death Dis. 2014;5(1):e997. [CrossRef]
- 56. Eiyama A, Okamoto K. PINK1/Parkin-mediated mitophagy in mam- malian cells. Curr Opin Cell Biol. 2015;33:95-101. [CrossRef]
- 57. Ge P, Dawson VL, Dawson TM. PINK1 and Parkin mitochondrial quality control: A source of regional vulnerability in Parkinson’s dis- ease. Mol Neurodegener. 2020;15(1):20. [CrossRef]
- 58. Fatokun AA, Dawson VL, Dawson TM. Parthanatos: mitochondrial- linked mechanisms and therapeutic opportunities. Br J Pharmacol. 2014;171(8):2000-2016. [CrossRef]
- 59. Wang YQ, Chang SY, Wu Q, et al. The protective role of mitochon- drial ferritin on erastin-induced ferroptosis. Front Aging Neurosci. 2016;8:308. [CrossRef]
- 60. Littlewood TD, Bennett MR. Apoptotic cell death in atherosclerosis. Curr Opin Lipidol. 2003;14(5):469-475. [CrossRef]
- 61. Franck G, Mawson TL, Folco EJ, et al. Roles of PAD4 and NETosis in experimental atherosclerosis and arterial injury: implications for superficial erosion. Circ Res. 2018;123(1):33-42. [CrossRef]
- 62. Bai T, Li M, Liu Y, Qiao Z, Wang Z. Inhibition of ferroptosis alleviates atherosclerosis through attenuating lipid peroxidation and endothe- lial dysfunction in mouse aortic endothelial cell. Free Radic Biol Med. 2020;160:92-102. [CrossRef]
- 63. Khalkar P, Díaz-Argelich N, Antonio Palop J, Sanmartín C, Fer- nandes AP. Novel Methylselenoesters induce programed cell death via Entosis in pancreatic cancer cells. Int J Mol Sci. 2018;19(10). [CrossRef]
- 64. Galluzzi L, Buqué A, Kepp O, Zitvogel L, Kroemer G. Immunogenic cell death in cancer and infectious disease. Nat Rev Immunol. 2017;17(2):97-111. [CrossRef]
- 65. Victor VM, Apostolova N, Herance R, Hernandez-Mijares A, Rocha M. Oxidative stress and mitochondrial dysfunction in athero- sclerosis: mitochondria-targeted antioxidants as potential therapy. Curr Med Chem. 2009;16(35):4654-4667. [CrossRef]
- 66. Yu LM, Dong X, Xue XD, et al. Melatonin attenuates diabetic car- diomyopathy and reduces myocardial vulnerability to ischemia- reperfusion injury by improving mitochondrial quality control: role of SIRT6. J Pineal Res. 2021;70(1):e12698. [CrossRef]
- 67. Parikh S, Saneto R, Falk MJ, et al. A modern approach to the treat- ment of mitochondrial disease. Curr Treat Options Neurol. 2009; 11(6):414-430. [CrossRef]
- 68. Zhang Y, Ren Y, Chen X, Deng S, Lu W. Role of butylphthalide in immunity and inflammation: butylphthalide may be a potential therapy for anti-inflammation and immunoregulation. Oxid Med Cell Longev. 2022;2022:7232457. [CrossRef]
- 69. Zhang X, Li G. Effects of a mitochondrial-acting drug on ischemia/ reperfusion-induced ventricular arrhythmias, cardiac mitochondrial function and inflammatory cytokines in rat: role of adenosine triphosphate-sensitive potassium channels. Eur J Inflamm. 2022;20:1721727X221115041. [CrossRef]
- 70. Morava E, Rodenburg R, van Essen HZ, De Vries M, Smeitink J. Dietary intervention and oxidative phosphorylation capacity. J Inherit Metab Dis. 2006;29(4):589. [CrossRef]
- 71. Tonkonogi M, Sahlin K. Physical exercise and mitochondrial function in human skeletal muscle. Exer Sport Sci Rev. 2002;30(3):129-137. [CrossRef]
- 72. Phielix E, Meex R, Moonen-Kornips E, Hesselink MK, Schrauwen P. Exercise training increases mitochondrial content and ex vivo mitochondrial function similarly in patients with type 2 diabetes and in control individuals. Diabetologia. 2010;53(8):1714-1721. [CrossRef]
- 73. Chausse B, Vieira-Lara MA, Sanchez AB, Medeiros MH, Kowal- towski AJ. Intermittent fasting results in tissue-specific changes in bioenergetics and redox state. PLOS ONE. 2015;10(3):e0120413. [CrossRef]
- 74. Liu H, Javaheri A, Godar RJ, et al. Intermittent fasting preserves beta-cell mass in obesity-induced diabetes via the autophagy- lysosome pathway. Autophagy. 2017;13(11):1952-1968. [CrossRef]
- 75. Godar RJ, Ma X, Liu H, et al. Repetitive stimulation of autophagy- lysosome machinery by intermittent fasting preconditions the myo- cardium to ischemia-reperfusion injury. Autophagy. 2015;11(9): 1537-1560. [CrossRef]
- 76. Moreira OC, Estébanez B, Martínez-Florez S, de Paz JAd, Cuevas MJ, González-Gallego J. Mitochondrial function and mitophagy in the elderly: effects of exercise. Oxid Med Cell Longev. 2017;2017: 2012798. [CrossRef]
- 77. Tam BT, Siu PM. Autophagic cellular responses to physical exercise in skeletal muscle. Sports Med. 2014;44(5):625-640. [CrossRef]
- 78. Broskey NT, Greggio C, Boss A, et al. Skeletal muscle mitochondria in the elderly: effects of physical fitness and exercise training. J Clin Endocrinol Metab. 2014;99(5):1852-1861. [CrossRef]
- 79. Gurd BJ. Deacetylation of PGC-1α by SIRT1: importance for skeletal muscle function and exercise-induced mitochondrial biogenesis. Appl Physiol Nutr Metab. 2011;36(5):589-597. [CrossRef]
- 80. Zhou Y, Wang S, Li Y, Yu S, Zhao Y. SIRT1/PGC-1α signaling pro- motes mitochondrial functional recovery and reduces apoptosis after intracerebral hemorrhage in rats. Front Mol Neurosci. 2017; 10:443. [CrossRef]
- 81. Cascella M, Bimonte S, Muzio MR, Schiavone V, Cuomo A. The efficacy of epigallocatechin-3-gallate (green tea) in the treatment of Alzheimer's disease: an overview of pre-clinical studies and translational perspectives in clinical practice. Infect Agent Cancer. 2017;12:36. [CrossRef]
- 82. Perez Ortiz JM, Swerdlow RH. Mitochondrial dysfunction in Alzhei- mer's disease: role in pathogenesis and novel therapeutic opportu- nities. Br J Pharmacol. 2019;176(18):3489-3507. [CrossRef]
- 83. Qi X, Qvit N, Su YC, Mochly-Rosen D. A novel Drp1 inhibitor dimin- ishes aberrant mitochondrial fission and neurotoxicity. J Cell Sci. 2013;126(3):789-802. [CrossRef]
- 84. Wang G, Yang Y, Ma H, et al. LncRNA FENDRR inhibits ox-LDL induced mitochondrial energy metabolism disorder in aortic endothelial cells via miR-18a-5p/PGC-1α signaling pathway. Front Endocrinol (Lausanne). 2021;12:622665. [CrossRef]
APA | Doğan N, coban n (2023). Mitokondriyal Disfonksiyonun Koroner Arter Hastalığı Üzerine Etkisi - Bölüm II. , 202 - 211. 10.5543/tkda.2022.07282 |
Chicago | Doğan Nazlı,coban neslihan Mitokondriyal Disfonksiyonun Koroner Arter Hastalığı Üzerine Etkisi - Bölüm II. (2023): 202 - 211. 10.5543/tkda.2022.07282 |
MLA | Doğan Nazlı,coban neslihan Mitokondriyal Disfonksiyonun Koroner Arter Hastalığı Üzerine Etkisi - Bölüm II. , 2023, ss.202 - 211. 10.5543/tkda.2022.07282 |
AMA | Doğan N,coban n Mitokondriyal Disfonksiyonun Koroner Arter Hastalığı Üzerine Etkisi - Bölüm II. . 2023; 202 - 211. 10.5543/tkda.2022.07282 |
Vancouver | Doğan N,coban n Mitokondriyal Disfonksiyonun Koroner Arter Hastalığı Üzerine Etkisi - Bölüm II. . 2023; 202 - 211. 10.5543/tkda.2022.07282 |
IEEE | Doğan N,coban n "Mitokondriyal Disfonksiyonun Koroner Arter Hastalığı Üzerine Etkisi - Bölüm II." , ss.202 - 211, 2023. 10.5543/tkda.2022.07282 |
ISNAD | Doğan, Nazlı - coban, neslihan. "Mitokondriyal Disfonksiyonun Koroner Arter Hastalığı Üzerine Etkisi - Bölüm II". (2023), 202-211. https://doi.org/10.5543/tkda.2022.07282 |
APA | Doğan N, coban n (2023). Mitokondriyal Disfonksiyonun Koroner Arter Hastalığı Üzerine Etkisi - Bölüm II. Türk Kardiyoloji Derneği Arşivi, 51(3), 202 - 211. 10.5543/tkda.2022.07282 |
Chicago | Doğan Nazlı,coban neslihan Mitokondriyal Disfonksiyonun Koroner Arter Hastalığı Üzerine Etkisi - Bölüm II. Türk Kardiyoloji Derneği Arşivi 51, no.3 (2023): 202 - 211. 10.5543/tkda.2022.07282 |
MLA | Doğan Nazlı,coban neslihan Mitokondriyal Disfonksiyonun Koroner Arter Hastalığı Üzerine Etkisi - Bölüm II. Türk Kardiyoloji Derneği Arşivi, vol.51, no.3, 2023, ss.202 - 211. 10.5543/tkda.2022.07282 |
AMA | Doğan N,coban n Mitokondriyal Disfonksiyonun Koroner Arter Hastalığı Üzerine Etkisi - Bölüm II. Türk Kardiyoloji Derneği Arşivi. 2023; 51(3): 202 - 211. 10.5543/tkda.2022.07282 |
Vancouver | Doğan N,coban n Mitokondriyal Disfonksiyonun Koroner Arter Hastalığı Üzerine Etkisi - Bölüm II. Türk Kardiyoloji Derneği Arşivi. 2023; 51(3): 202 - 211. 10.5543/tkda.2022.07282 |
IEEE | Doğan N,coban n "Mitokondriyal Disfonksiyonun Koroner Arter Hastalığı Üzerine Etkisi - Bölüm II." Türk Kardiyoloji Derneği Arşivi, 51, ss.202 - 211, 2023. 10.5543/tkda.2022.07282 |
ISNAD | Doğan, Nazlı - coban, neslihan. "Mitokondriyal Disfonksiyonun Koroner Arter Hastalığı Üzerine Etkisi - Bölüm II". Türk Kardiyoloji Derneği Arşivi 51/3 (2023), 202-211. https://doi.org/10.5543/tkda.2022.07282 |